Catalyst-impregnated ceramic filter elements can achieve simultaneous removal of multiple air-pollutant species in a modular, space-saving layout
The three most common pollutants found in the air we breathe are particulate matter (PM), nitrogen oxides (NOx) and sulfur oxides (SOx). These three major sources of pollution can trigger respiratory difficulties and asthma, as well as cause environmental harm in the forms of acid rain, visibility impairments and water-quality impacts. The U.S. Environmental Protection Agency (EPA; Washington, D.C.; www.epa.gov) regulates all three pollutants, which may be emitted in fluegas from coal-fired power plants, fertilizer plants, glass manufacturing plants, cement plants, petroleum refineries and other industrial facilities. The EPA manages a comprehensive enforcement and compliance database of thousands of industrial plants in the U.S. via the Enforcement and Compliance History Online (ECHO) system (echo.epa.gov).
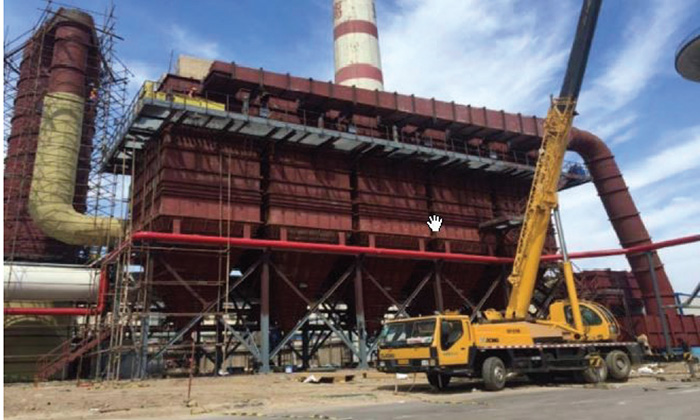
FIGURE 1. Multiple pollutants in fluegas can be effectively treated with ceramic catalytic filter (CCF) elements. This system shows five installed filter boxes
Currently, industrial pollution-control equipment installations are dominated by baghouse fabric filters and electrostatic precipitators (ESPs). Simultaneous removal of the three major pollutants from fluegas can also be achieved with catalyst-impregnated ceramic filter elements within one housing-boxed system (Figure 1). Such catalyst ceramic filtration (CCF) installations can achieve simultaneous pollutant removal with lower capital expenditure and plot-space requirements than traditional baghouse or ESP configurations. Figure 2 summarizes the removal efficiencies of a CCF unit for various pollutants. CCF systems have seen successful installations in many industrial applications, including the following:
- Hazardous waste incineration
- Industrial waste incineration
- Glass industry furnaces (float glass, tableware and container)
- Biomass energy plants
- Synthesis gas (syngas) processing
- Regenerative thermal oxidizer exhaust
- Ceramic kiln exhaust
- Catalyst manufacturing
- Munitions incineration
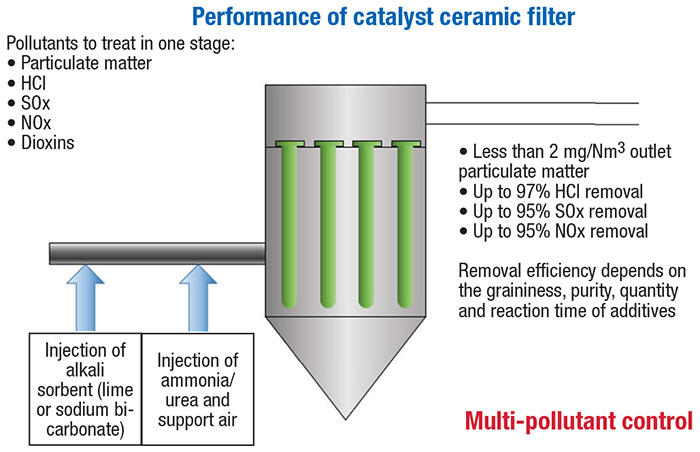
FIGURE 2. A CCF system can simultaneously remove multiple pollutants, including SOx, NOx, particulate matter and dioxins
Tackling air pollution
To understand the process specifics for CCF systems, it is first important to outline the traditional treatment technologies for the major air pollutants: NOx, SOx and PM. These methods are described in the following sections.
NOx treatment. Oxides of nitrogen (NOx) include nitrogen dioxide and nitric oxide. Common technologies used to remove NOx are selective catalytic reduction (SCR), which reacts NOx with ammonia at around 700°F with the aid of a catalyst, and selective non-catalytic reduction (SNCR), which reacts with ammonia at even higher temperatures to reduce NOx emissions. Catalytic reduction of NOx is typically performed at a temperature range between 600 and 700°F. The NOx-reduction operation is performed by injecting an ammonia-based reagent.
In applications with high-temperature furnace offgas (around 1,150°F), water spray injection, fresh air dilution or heat exchangers can be used to cool and control the gas velocity and temperature distribution in order to reach the optimum reaction conditions.
In order to avoid the risk of undesired formation of ammonium sulfate (AS) or ammonium bisulfate (ABS) at lower temperatures, the inlet temperature must be controlled to remain above 662°F. The ammonia-based gas thus created then reacts with the NOx over a catalyst. Catalysts typically consist of TiO2, V2O5 or WO3. The main reactions occurring in catalytic NOx treatment are as follows:
NO + NO2 + 2NH3 ➝ 2N2 + 3H2O (fast)
4NO + 4NH3 + O2 ➝ 4N2 + 6H2O (slow)
2NO2 + 4NH3 + O2 ➝ 3N2 + 6H2O (slow)
SOx treatment. Sulfur oxides (SOx), mainly SO2, are of concern due their potential to create sulfuric acids. For SOx removal, the commonly used equipment are wet fluegas desulfurization (WFGD), the circulating dry scrubber (CDS) and the semi-dry scrubber (SDA). In FGD, lime reacts in operating temperature ranges between 350 and 1,200°F with SO2 in order to form salt and water through the following reaction, where calcium hydroxide (hydrated lime) and sodium-based sorbents are used, such as sodium bicarbonate or Trona (trisodium hydrogendicarbonate dihydrate):
Ca(OH)2 + SO2 + ½O2 ➝ CaSO4 + H2O
Processed PM and neutralization residues are then transported through a pneumatic conveying system to an intermediate storage silo or to the batch house for reuse.
Acid-gas neutralization is performed via the injection of high-specific-area hydrated lime in the duct upstream of the catalyst-embedded ceramic filters with a dry sorbent injection (DSI) technology. The contact between acid gases and hydrated lime particles is increased because the outgoing gases must pass through the filtration cake layer containing the hydrated lime deposited on the outer surface of the CCF element.
PM treatment. PM comprises a mixture of solid particles, liquid droplets, dust, dirt, soot and smoke (ranging from 2.5 to 10 microns [µm] in size). The mixture can contain varied components, such as bases, acids, other chemicals, liquids, solids, metal dust and gas particles, which are commonly removed using a baghouse fabric filter or a wet or dry ESP. The EPA regulates PM content through established air-quality standards based on particle size, including PM2.5 regulations (related to fine particles less than 2.5µm in diameter) and PM10 regulations (related to particles less than 10µm in diameter).
Multi-pollutant control
As previously mentioned, there are several common methods (including SCR, SDA and baghouses) found in industry to remove NOx, SOx and PM. Depending on the application, there are various types of equipment used in such air-pollution-abatement technologies. These are summarized in Table 1. Technology selection will depend on application needs and best available technologies (BAT).
Cost-comparison studies have been completed to compare one system combination of equipment with other combinations for various industrial applications. An in-depth study undertaken by the Economic Commission for Europe [1] provides comprehensive information on selection of air-pollution-control methods.
A primary benefit of utilizing CCF configurations is to reduce the amount of equipment being used and hence reduce the onsite footprint, as shown in Figure 3. Other benefits of CCF units include their resistance to high temperatures and corrosion, long lifecycle (5- to 10-year operating life of the catalyst filter elements), high heat-recovery efficiency and relatively low operating costs.
Fabric filters (also called baghouses) and ceramic filters can capture over 99.9% of particulate matter, including PM2.5. These become multi-pollutant systems when combined with sorbent injection (as shown in Figure 3) to remove additional pollutants, such as mercury, SOx and other acid gases. NOx can be removed via SCR catalysts incorporated into the filter elements and upstream injection of ammonia or, if the temperature is sufficiently high, urea.
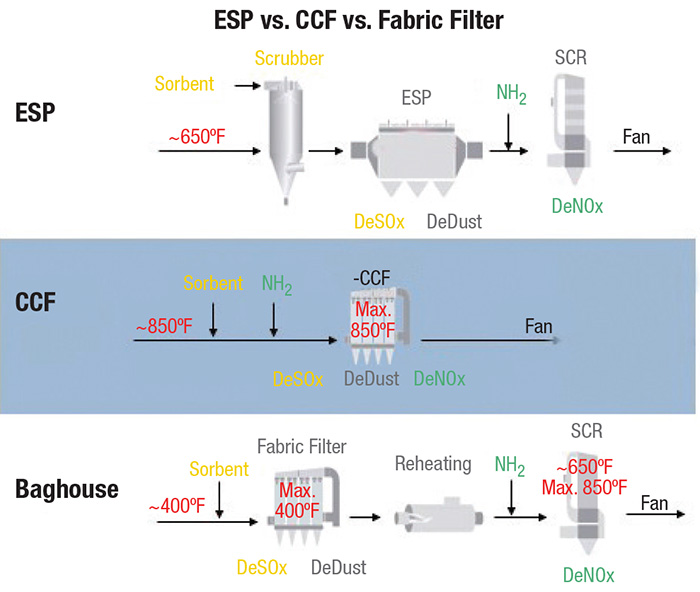
FIGURE 3. CCF installations have a more compact equipment layout than other air-pollution-control technologies, such as fabric baghouse filters or electrostatic precipitators (ESP)
Ceramic filters are also called candle filters because of their solid tube shape. Low-density ceramic filters can be provided with or without an embedded catalyst.
The use of CCF box systems allows for multi-pollutant control in one step. Beyond their smaller footprint for multi-pollutant removal of SOx, NOx and PM, CCF systems have also been shown to remove dioxins and heavy metals. Furthermore, CCF installations, due to their smaller footprint, typically have a lower pressure drop across the system, and require less maintenance and consume fewer utilities than other pollution-control technologies. CCF systems can run at high exhaust-gas temperatures (greater than 350ºF) and typically operate far away from acid and water dewpoint temperatures. Their modular nature means that one filter box can be maintained individually without interrupting operation. Filter cake removal is achieved by automatic online pulse-jet cleaning.
Ceramic filter fabrication
Candle-shaped ceramic filters are rigid tubes, with 80 to 90% porosity and lightweight refractory fibers, plus organic and inorganic binding agents (Figure 4). Dispersant agents, pore-forming agents, high-temperature binders and plasticizers are typically added to produce the filter tube, allowing for excellent thermal resistance and thermal shock resistance. The fibers’ structure enables a high internal surface area. The filters are most commonly supplied in 10-ft lengths and 6-in. diameters and typically weigh around 12.5 kg (27 lb). Figure 5 shows typical dimensions and structure. Some suppliers are investigating even longer lengths of 20 ft, allowing for fewer elements per box, resulting in even smaller footprints.
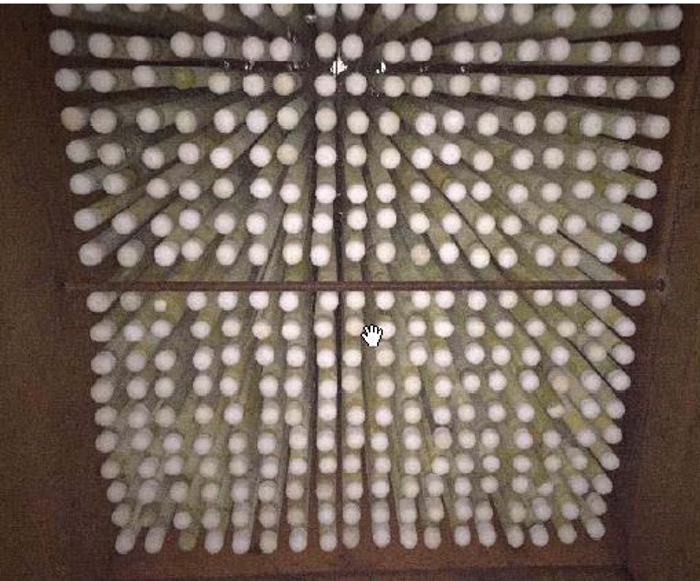
FIGURE 4. Tubular filter elements imbue CCF installations with high internal surface area
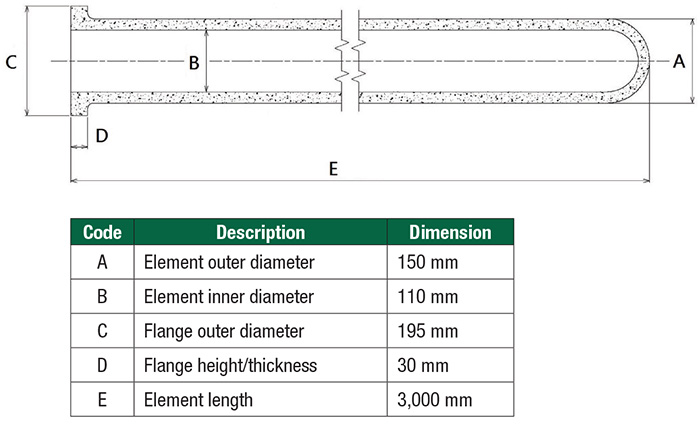
FIGURE 5. This diagram shows typical dimensions for CCF structures
Low-density ceramic filters made up of ~3-µm dia. fibers are vacuum-formed into a rigid, tube-shaped fibrous filter element from a fiber slurry. The filter elements are installed as an alternative to conventional pulse-jet-cleaned fabric-filter elements. The filter elements are self-supported via an integral flange, as shown in Figure 6. The pressure drop across the filter boxes are held at around 4 in. H2O(g) when in a clean condition.
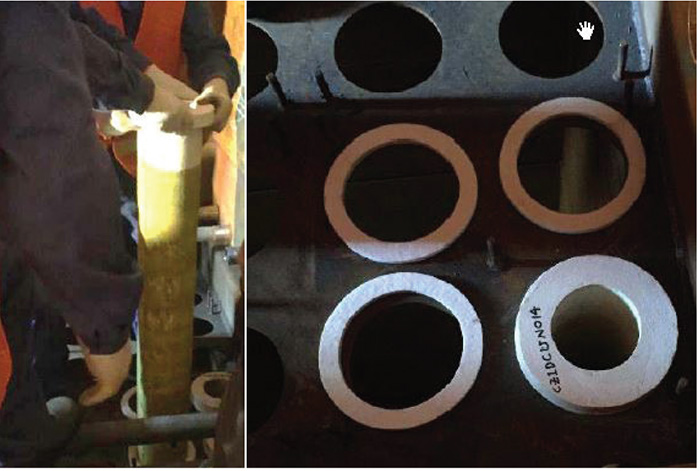
FIGURE 6. Filter elements are self-supported with an integral flange
Dry calcium- or sodium-based sorbents are injected into the fluegas upstream of the ceramic filter for SO 2 removal. Activated carbon or brominated activated carbon could also be injected to remove mercury. The fluegas is drawn through the filter tube wall by an induced-draft (ID) fan, where the collected particulate matter will build up as a cake on the outside of the tube (Figure 7). NOx is removed by catalytic reduction with injected ammonia or urea to form nitrogen and water. The catalyst is embedded within the filter walls. The clean fluegas then passes up the tube into the plenum. The cake is periodically cleaned from the filter walls inline using standard pulse-jet methods. Optimum pulse-jet frequency and pressure improve NOx reduction.
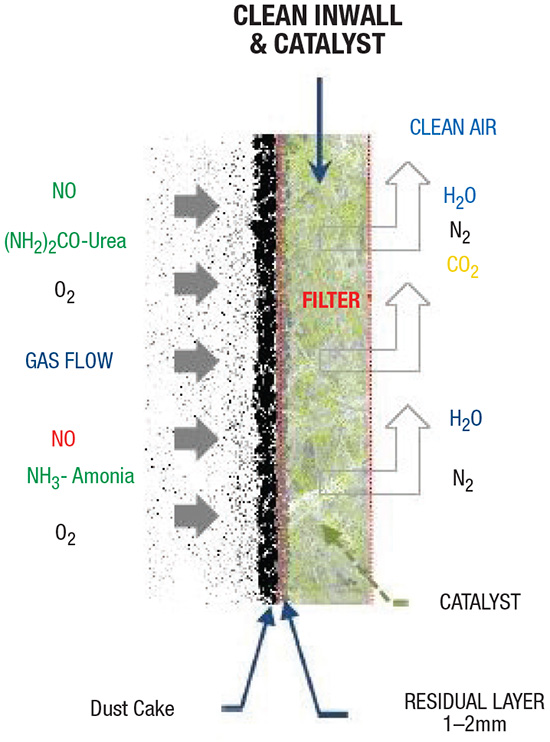
FIGURE 7. Particulate matter is removed from fluegas and collected inside the filter tube wall, while NOx is catalytically reduced using injected ammonia or urea. Sorbents injected upstream of the filter inlet mitigate SOx content
The ceramic filters are spray-embedded and dried with a catalyst slurry to facilitate reactions to remove NOx. The catalytic filter element consists mostly of Al2O3, SiO3, TiO2, V2O5 and WO3. The micrometer-sized catalyst particles are distributed across the entire wall thickness using automatic equipment, thus creating a large, stable catalytic surface area. The catalyst particles’ microporous structure and small size partially account for the increased reactivity at lower temperatures. To ensure the effectiveness of the catalytic particles, raw material inspection is crucial, as is robust sampling to check the catalytic activity after embedding.
CCF systems have a small footprint and are simple to operate. They could replace an existing ESP and eliminate the need for a separate SCR unit. Therefore, they can be retrofitted into industrial plants where there is not enough space to fit a conventional SCR unit.
The modular design of the boxed-housing units allows filters to be configured to handle large gas-flow volumes. The systems can be designed so that a single box can be taken offline if required, and the remaining two or more boxes continue to operate at a slightly higher pressure without interruption of the process itself and with no appreciable change in emissions.
No water is consumed in the process, and no wastewater is produced. However, the produced flyash residue requires disposal. Disposal of catalytic ceramic filters is required when they reach the end of their life. The catalyst lifetime has been found to be higher than in high-dust conventional SCR systems. The typical life of ceramic filters is five to ten years, with some installations running even longer. Generally, visual inspection for cracks, holes or missing corners can indicate whether a filter element is damaged or needs replacement. However, if the overall appearance of the filter element is normal, but the pressure drop increases and de-nitration efficiency decreases, this is an indicator that the catalyst is no longer effective and emissions requirements cannot be achieved.
Future work
Although they have been successfully deployed across many industrial sectors, CCF systems have not yet been used widely in high-dust applications, such as those found in coal-fired power plants and cement plants. Recently, more studies have been done with CCF technologies to evaluate their performance in coal-fired plants that have high ash or dust content. The research work in Ref. 2 covers a pilot study that used actual fluegas from coal combustion to remove SOx, HCl, NOx and dust in a single step. The project achieved 95% NOx removal, 85% SOx removal and 91% HCl removal. Also, the research found that ceramic candle filters were stable against high temperatures, as well as the presence of acid gas content and particulate matter. This work proposes that ceramic catalytic filters could provide a novel and feasible approach to multipollutant control in high-dust applications, such as coal-fired power plants. As additional installations are completed, more and more applications will find benefits in the use of CCF systems for air-pollution control. ■
Edited by Mary Page Bailey
References
1. Economic Commission for Europe, Document ECE.EB.AIR.117_AV, Guidance document on control techniques for emissions of sulfur, nitrogen oxides, volatile organic compounds and particulate matter (including PM10, PM2.5 and black carbon) from stationary sources, Jan. 2015.
2. Zengqiang, T., Guoping N., others, Ultralow Emission of Dust, SOx, HCl, and NOx Using a Ceramic Catalytic Filter Tube, Energy Fuels, The American Chemical Society, March 2020.
3. Carpenter A. M., Advances in Multi-Pollutant Control, IEA Clean Coal Centre, Nov. 2013.
4. Heidenreich, S., Hot Gas Filtration — A Review, Fuel, Vol. 104, February 2013, pp. 83–94.
5. Moss, K., Advanced Multipollutant Control with Lightweight Ceramic Filters, Air Pollution Control, June 2011, pp. 15–19.
6. Moss, K., Ceramic Filter Systems, Ceramic Industry, June 2012, pp. 23–27.
7. Moss K., Advanced Air Pollution Control in One System, Pollution Engineering, Jan. 2012, pp. 12–16.
8. Startin A. and Elliott, G., Controlling Emissions with Ceramic Filters, Chem. Eng., Jan. 2009, pp. 35–39.
Authors
Mughis Raza Naqvi is a marketing consultant for FLKCAT Ltd. (Email: [email protected]; Website: www.cat-filter.com), a filtration and air-pollution specialist represented in the U.S. by Keying Enterprise. Naqvi has over 25 years of experience in the process equipment industry, serving the chemicals, glass, cement and energy sectors. He also has over 10 years of experience in air pollution control and water and wastewater treatment applications. His previous work experience includes environmental roles at Dürr Systems, Eisenmann Corp. and Lechler USA Inc. Naqvi holds a B.S.Ch.E. from McGill University and a graduate degree in business administration from Benedictine University. He is a licensed professional engineer in the province of Ontario, Canada.
Chung Lee is the general manager of FLKCAT Ltd. He holds a B.S. in environmental engineering from the National Sun Yat-sen University in Taiwan, and M.S. and Ph.D. degrees in chemical engineering from the New Jersey Institute of Technology. He also earned a master’s degree in law from Soochow University in Taipei, Taiwan. Chung holds a professional engineer license from the Chinese Institute of Environmental Engineering and the Taiwan Institute of Chemical Engineers. He has more than 25 years of experience in air-pollution control and wastewater treatment and wastewater recovery.
Amos Wang is the senior manager of the research and development department of FLKCAT in Kaohsiung City, Taiwan. He received a M.S. degree from the environmental engineering department of National Chung Hsing University in Taiwan, and previously served as the section manager of AAF International (American Air Filter) Taiwan with special focus on HEPA filtration.