The winning technology for the 2019 Kirkpatrick Chemical Engineering Achievement Award, along with the other finalist technologies, demonstrate how innovative engineering can tackle climate change and other challenges. All six technologies are described here
The six finalist technologies for the 2019 Kirkpatrick Chemical Engineering Achievement Award — including the winner, LanzaTech Inc.’s (Chicago, Ill.; www.lanzatech.com) gas-fermentation technology — represent the breadth of fields impacted by the chemical engineering profession. From industrial catalysis and petroleum refining to fermentation and bio-based plastics, the diverse set of technologies that garnered honor awards underlines the multidisciplinary nature of modern chemical engineering, and highlights the creativity and persistence of teams from across the globe.
The Kirkpatrick Award, given by Chemical Engineering magazine every other year since 1933, recognizes achievements in commercializing noteworthy chemical engineering technology. For a full list of previous award winners, see www.chemengonline.com/kirkpatrick-award/. The 2019 winning technology from LanzaTech, along with the five other finalist technologies — from Dow, Reliance, JohnsonMatthey, TechnipFMC and Braskem — are described here, along with some notes about recent progress.
2019 Award winner
LanzaTech Gas fermentation
In traditional fermentation, microbes metabolize sugars to generate chemical compounds, such as alcohols. LanzaTech has developed a process featuring microbes that feed on carbon-rich gases, including exhaust gases, rather than sugar, and convert them into ethanol.
“The way we procure, use and dispose of carbon is fundamental to the wellbeing of our planet,” says Allan Gao, senior process engineer at LanzaTech, “and we look forward to seeing more carbon recycling platforms deployed around the world.”
Gao continues: “We’re honored to receive the Kirkpatrick Award, and we appreciate the visibility of an award like this to highlight how technology and innovation can tackle climate change” (Figure 1).
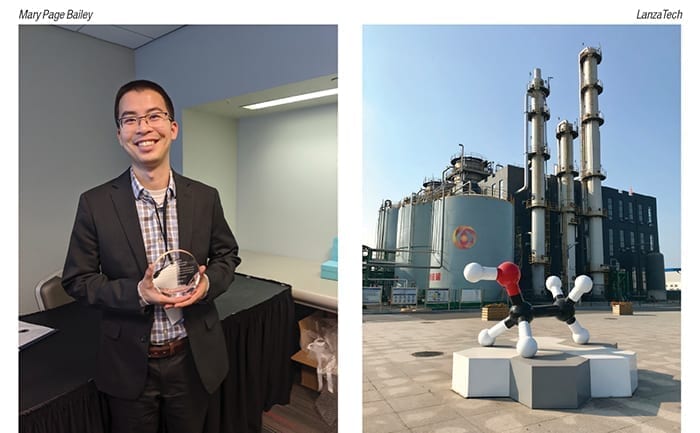
Figure 1. LanzaTech’s Allan Gao accepts the 2019 Kirkpatrick Award at the Chem Show in New York (left). A model of an ethanol molecule decorates the first commercial facility using LanzaTech’s gas-fermentation process, a steel mill in China (right)
The LanzaTech process offers an alternative to the Fischer-Tropsch (F-T) process, which has been used for decades to convert low-molecular-weight hydrocarbons that would otherwise be flared into liquid transportation fuels. F-T begins with synthesis gas from the gasification of hydrocarbons, coal or biomass. F-T has significant limitations, however, including the need for high pressures and the requirements that the synthesis be treated to remove diluents such as N2 and CO2, and remove contaminants that can poison the F-T catalysts. LanzaTech’s process occurs at low pressures (less than 10 bars) and can operate on a wide range of H2-to-CO ratios, converting the syngas to ethanol, which can then serve as a building block for other chemicals, such as rubber, plastics and fuels. Third-party assessments of the LanzaTech technology have shown greenhouse-gas-emissions reductions of over 70% compared to equivalent products from fossil carbon.
“Focus has been increasing on the use of non-food feedstocks to produce fuels, particularly in Europe and India,”explains Gao. “In addition to reducing greenhouse gas emissions, producing ethanol from industrial off-gas and waste positively impacts the environment in other ways. Also, the feedstock does not require land or additional resources to produce.”
Microbes capable of utilizing CO, known as acetogens were first discovered in 1903, but had not been exploited in a commercial process prior to LanzaTech’s work. The company was able to optimize the bacterium Clostridium autoethanogenum to produce ethanol at high selectivities from a wide range of gas feedstocks. LanzaTech’s Gao says ethanol selectivities as high as 95% can be achieved stably while operating with feed gas of fluctuating composition. “The ability of the process to tolerate variable gas feeds is critical for utilizing these off-gas and syngas feedstocks,” he says, which can come from steel-making and gasification of municipal solid waste and biomass.
Aside from the microbe, another development in the process was LanzaTech’s bioreactor, which solves two unique challenges of gas fermentation. First, the bioreactor design enables high gas-to-liquid mass transfer of CO and H2 at low pressure, “a critical requirement to enabling a cost-efficient high rate of reaction despite the low solubility of CO and H2 in aqueous solutions,” LanzaTech says. And second, the bioreactor is designed for concurrent consumption of CO and H2.
LanzaTech and its industrial partners had been working to scale-up and commercialize the gas fermentation process since 2005, resulting in more than 100,000 hours of pilot- and demonstration-scale operating data at five sites co-located at industrial sites and operating on real industrial gases and syngas, Gao says. The pilot and demonstration experience led to the May 2018 startup of the first operating commercial gas-fermentation facility in the world: the Shougang LanzaTech commercial plant at the Jingtang Steel Mill in Caofeidian, Hebei Province, China.
The commercial plant in China has produced more than 10 million gallons of ethanol since startup in 2018. Ethanol from the plant is being used as a transportation fuel, but is also being converted into products, such as high-density polyethylene (HDPE) and ethylene glycol for use in the manufacture of polyethylene terephthalate (PET) plastic.
Plans are underway for the LanzaTech process to be deployed at other commercial sites, involving steel mill off-gas, refinery off-gas, and biomass and municipal solid waste syngas feedstocks. “The next two commercial plants in the pipeline are with Indian Oil Corp. and ArcelorMittal, operating on refinery off-gas and steel mill off-gas respectively, with the intent to construct further plants across their operations,” says Gao.
LanzaTech is developing a number of downstream conversion routes for the use of the ethanol, including conversion to jet fuel, HDPE and a recently announced collaboration to convert ethanol into ethylene glycol for use in a high-tenacity yarn product, Gao says.
Converting alcohol to jet fuel
LanzaTech recently announced progress in scaling up its alcohol-to-jet (ATJ) platform. The company entered a partnership with the U.S Department of Energy’s Pacific Northwest National Laboratory (PNNL; Richland, Wash.; www.pnnl.gov) in which PNNL developed a unique catalytic process to upgrade ethanol to alcohol-to-jet synthetic paraffinic kerosene (ATJ-SPK) and LanzaTech scaled up the process from laboratory to pilot scale.
After the April 2018 scaleup, ethanol was added as an approved feedstock in ASTM D7566 Annex A5 (Standard Specification for Aviation Turbine Fuel Containing Synthesized Hydrocarbons for ATJ-SPK), and in October 2018, it was used to power a commercial flight with Virgin Atlantic.
Now plans are underway to build a demonstration-scale facility for the jet fuel made ultimately from captured exhaust. Michael Berube, DOE deputy assistant secretary for transportation, announced that DOE is negotiating with LanzaTech for a $14 million investment in a demonstration-scale integrated biorefinery at LanzaTech’s Freedom Pines site in Soperton, Ga.
The PNNL/LanzaTech ATJ process can use any source of sustainable ethanol for jet fuel production, including ethanol made from recycled pollution. The flexibility of the technology to utilize a variety of local waste feedstocks attracted the attention of Japan’s All Nippon Airways (ANA), resulting in an offtake agreement with LanzaTech signed in 2019, allowing ANA to purchase sustainable aviation fuel from LanzaTech’s process.
Following this agreement, ANA, Mitsui & Co. and JXTG Energy have been selected by the New Energy and Industrial Technology Development Organization (NEDO; a Japanese public research organization) to conduct a feasibility study on scaling the LanzaTech ATJ platform in Japan. The partners aim to establish a sustainable domestic supply chain for ATJ, key to achieving full commercial deployment in Japan.
ANA and Mitsui & Co. kicked off the project by conducting a Boeing 777-300ER ferry flight using sustainable aviation fuel made from recycled carbon on October 30, 2019. As the fuel producer, LanzaTech worked closely with all partners, advising how best to transport and blend the fuel for loading on the aircraft.
“Sustainable aviation fuel reduces carbon emissions by up to 80% and is a key element of the industry’s climate action strategy,” says Sheila Remes, vice president of strategy at Boeing Commercial Airplanes. “ANA’s flight demonstrated that sustainable fuel blends with conventional fuel without the need for any changes to the airplane, engines or airport fueling infrastructure.”
LanzaTech’s carbon capture platform for sustainable aviation fuel is now poised for scale up in the U.S. and Japan. In the U.K., LanzaTech is a shortlisted applicant for a grant from the U.K. Department for Transport (DfT) through the Fuels for Flight and Freight Competition (F4C). This grant would support deployment of the technology in the U.K.
2019 honor awards
JohnsonMatthey Catacel SSR
Most hydrogen for industrial use in petroleum refining, ammonia production and other applications is made via steam-reforming of natural gas over catalyst-impregnated ceramic pellets. Recent increased demand for hydrogen has pushed operators of steam methane reformers (SMRs) to maximize capacities, however, doing so can often lead to limitations in temperature, pressure and feed flows.
The current design of catalyst pellets improves catalyst activity and heat transfer, but further improvement is blocked as gains come with higher pressure drop. To boost catalyst performance, Johnson Matthey (JM; London, U.K.; www.matthey.com) has developed an innovative alternative to pellets: CATACEL SSR, a nickel-catalyst-coated metal foil technology that has been proven to increase catalyst activity and heat-transfer while simultaneously decreasing pressure drop by up to 20%. In fact, increases of 15–20% have been observed in plant throughput rates.
CATACEL SSR are designed to replace catalyst pellets in existing reformer tubes without the need for new capital investment. The structured foil units use alloy strips, engineered into a series of V-shaped bends (Figure 2), that the company calls fans, onto which the catalyst material is coated. The fans are stacked into mechanical assemblies that fit inside of the reformer tube. JM’s proprietary catalyst coating technology allows the catalyst to durably attach to the fans’ thin metal foil surface covering a high surface area. The stacked fans deliver superior heat transfer by intentionally jetting the gas on the internal surface of the reforming tube, rather than relying on the random flow of gas through a pellet bed. CATACEL SSR technology for steam-methane reformers enables gains in process performance that are not achievable with conventional pellet catalyst technologies, JM says.
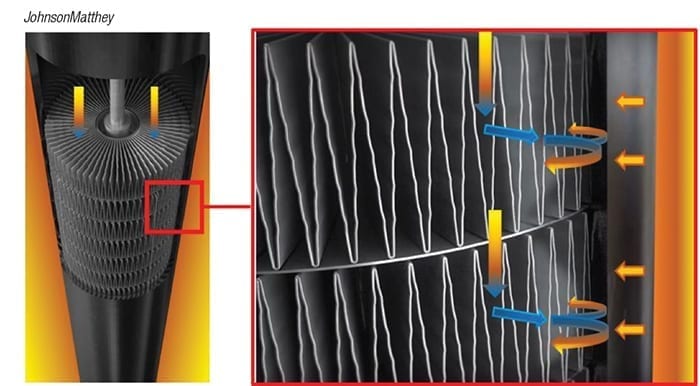
Figure 2. A novel catalyst for the steam-reforming of methane developed by JohnsonMatthey uses strips of alloy material folded and coated with catalyst material to improve heat transfer and reduce pressure drop
Dow Symbiex
Flexible food packaging often uses different polymer materials layered together to form laminates that exhibit the full range of properties required for mechanical performance and food-contact regulations. Lamination adhesives are used to bind the layers together. According to Dow Packaging & Specialty Plastics (Dow; Midland, Mich.; www.dow.com), the trend has been toward faster-curing adhesives because they allow operational advantages (faster time to market and lower warehousing costs). Dow’s Symbiex technology speeds the curing times for multilayer packaging.
Developed along with machinery manufacturer Nordmeccanica NA (Hauppauge, N.Y.; www.nordmeccanica.com), Dow’s Symbiex overcomes a major challenge for plastic laminate adhesives, which is to balance the need for fast curing with the need to maintain easy processing.
The “pot life” of an adhesive refers to the time it takes for the mixed adhesive to increase its molecular weight to a point where it compromises the quality of the laminate in which it is used. For practical processing, it is desirable to have a long pot life, so operators can stop and start the machine between jobs without risking product quality. But once the adhesive is applied in the laminate, the imperative switches to fast curing time.
The new lamination concept effectively separates the two components of the adhesive until they are actually together within the laminate. The cure only begins when the two coated films are brought together to form the multilayer film, and not before, so a convenient pot life is maintained, while a fast cure is achieved once the adhesive components join with in the plastic laminate, Dow says.
To make the system work, Dow and Nordmeccanica had to rethink and redesign the lamination machinery (Figure 3), while also developing modifications to the adhesive chemistry. The final system achieved improved pot life (4–6 h versus 20 min for conventional adhesive technology), as well as accelerated curing time (less than one day versus 5–8 days with conventional technology.
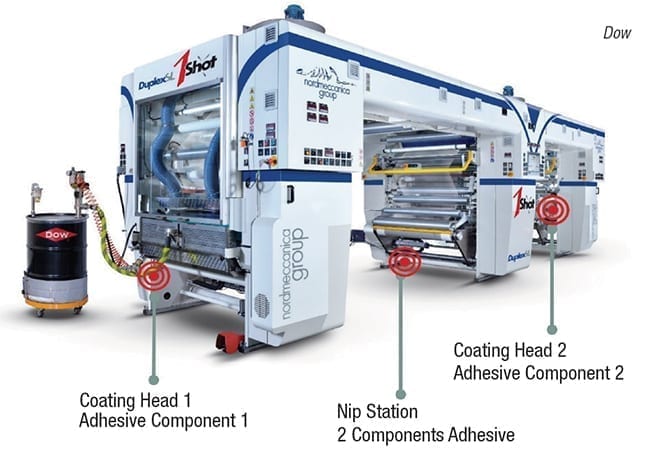
Figure 3. To allow for fast cure times in plastic laminate adhesives, the Dow Symbiex technology keeps the adhesives apart begins the cure phase only when the components of the laminate are joined
TechnipFMC Direct-Heating Unit
The TechnipFMC (Houston; www.technipfmc.com) Direct Heating Unit (DHU) is a groundbreaking technology for adding heat to high-temperature processes. Unlike conventional furnace burners, where fuel and air are combined at a single point and a flame is present, fuel in the DHU is added incrementally to a high-velocity air stream via multiple injections over an extended reaction zone. As a result, the fuel reacts in a controlled manner at significantly lower temperatures than classic combustion and without a visible flame.
The concept of flameless distributed combustion was developed in the 1990s by Shell Oil for use in wellbore heaters for enhanced oil recovery. TechnipFMC licensed the technology from Shell for further development and application of the technology to process heating in styrene manufacturing.
The DHU consists of multiple sets of four concentric tubes, where a fuel-containing tube is the innermost and is surrounded by a reaction tube where the fuel and air combine. The fuel flows outward into the reaction zone through a precise pattern of orifices arranged along the length of the process-heating zone (Figure 4). Since the reaction air is pre-heated in the air tube, which Is the third concentric tube, by contact with the reaction tube, it enters the reaction tube at temperatures above the autoignition temperature of the fuel, which also has been pre-heated. Air and fuel are mixed and reacted continuously between the reaction tube and fuel tube, and the products flow up to the exhaust channel. The process fluid enters the DHU through a side nozzle and flows downward in the annular space between the outside of the air tube and the inside of the process sleeve, which is the fourth and outer most concentric tube. The process feed absorbs the heat released by the reacting fuel through both convection and radiation.
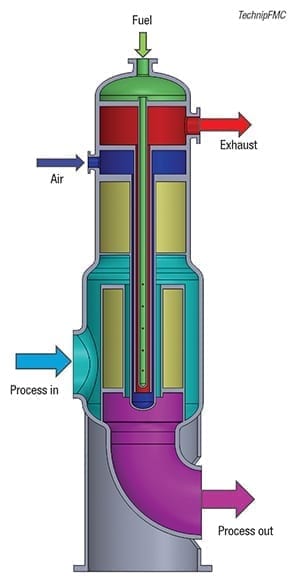
Figure 4. The Direct Heating Unit (DHU) uses flameless combustion, and is safer than a traditional fired burner system, with less emergency shutdowns, according to the developer TechnipFMC
TechnipFMC says the DHU is far safer to operate than a traditional fired heater and has fewer emergency shutdown switches. In addition, the use of a DHU in styrene manufacturing reduces capital cost requirements, enables significant reductions in energy consumption in the process, and is operated more like a heat exchanger than a fired heater.
Along with Total Petrochemicals, TechnipFMC installed a DHU demonstration unit at Total’s Cosmar Styrene Plant in Carville, La. After meeting the objectives for the DHU demonstration unit in over 10,000 hours of operation, a full-scale DHU was installed at an existing styrene production plant in Truinfo, Brazil. Videolar-INNOVA S.A. (INNOVA) recently started up the DHU at INNOVA’s styrene plant, the first commercially operating example of the DHU technology in the world. INNOVA industrial director Sergio de Oliveira Machado states, “The DHU technology has surpassed our expectations and we are very pleased with how the project was completed with full cooperation from TechnipFMC’s Badger Technology.”
A second commercial-scale application of the DHU technology has also started up, TechnipFMC says.
The DHU technology was jointly developed with Total Petrochemicals and Shell Catalysts, and Technologies, and is licensed through TechnipFMC’s Badger Process Technology Group. DHU technology is now routinely incorporated as part of the Total/Badger styrene technology for grassroots and revamp applications, TechnipFMC says.
Reliance benzene/gasoline
U.S. EPA regulations restrict the average benzene level in gasoline to 0.62%. To meet the requirement, Reliance Industries Ltd. (RIL; Ghansoli, India; www.ril.com) and CSIR Indian Institute of Petroleum (Dehradun, India; www.iip.res.in) jointly developed the world’s first technology for processing the C6 heart cut of FCC (fluid catalytic cracking) gasoline. The technology is based on the principles of extractive distillation and does not need a pre-processing step to saturate di-olefins and reduce reactive impurities (chlorides, oxygenates, metals and others). The method not only produces EPA-compliant gasoline but also recovers high-purity benzene.
The technology features the most thermally and chemically stable and tunable solvent system for handling reactive impurities in FCC gasoline, along with an innovative and proprietary process configuration that minimizes solvent loss and utility requirements while maximizing product purity and yield.
The first commercial unit using this technology operates at RIL’s petrochemical complex in Jamnagar, India (Figure 5). The benzene content of the raffinate (gasoline) has been consistently below 0.05%, while benzene recovery has exceeded 99% while producing extract with more than 98% benzene, RIL says. Patents for the technology have been granted in several countries.
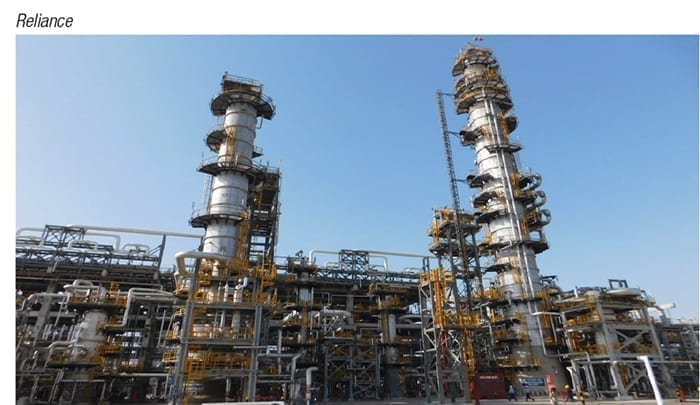
Figure 5. Benzene levels in FCC gasoline at the Reliance refinery in Mumbai have dropped to 0.05% and benzene recovery is 99%
Braskem renewable EVA
Braskem (Sao Paulo, Brazil; www.braskem.com.br) developed a process for renewable ethylene vinyl acetate (EVA) along with the footwear company Allbirds Inc. where sugarcane, instead of oil, was used as the raw material. Conventionally, EVA is produced from petroleum-derived ethylene. With input from a number of sources, Braskem developed a new resin to produce a range of EVA and EVA rubber products in a plant originally designed to produce ethylene. The first commercial product to use the new EVA is a line of flip-flops.