The infrared approach to temperature monitoring is especially useful in situations where process temperatures are extremely high, the target is in motion, and the need to avoid contaminants is significant
Accurate temperature measurement can often be quite challenging, especially in situations where process temperatures are extremely high (above 800°F), the target is in motion, or the need to avoid contaminants is significant. Infrared (IR) temperature-sensing offers an attractive solution.
IR devices function basically by measuring infrared energy at a distance. Infrared energy is emitted by all objects directly at quantities that relate to their host object’s temperature. Waves pass through the sensor optics, are converted to electrical signals at the detector and then are displayed as a temperature.
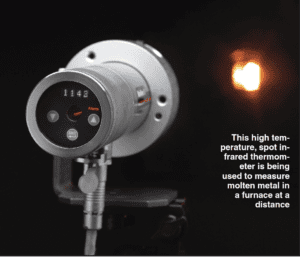
This high temperature, spot infrared thermometer is being used to measure molten metal in a furnace at a distance
THE ADVANTAGE OF IR
Infrared sensors offer advantages over many other types of temperature sensors:
Non-contact. They do not need to touch an object to detect it’s temperature.
Rapid response. They can measure infrared energy and reinterpret the waves as temperature data in milliseconds. Most mechanical or resistance-based thermometers function instead by placement within and adapting to the temperature of the environment or object being measured in order to provide a reading. These devices require more wait time than infrared devices.
High precision. They can measure an object’s energy output precisely, and therefore detect it’s temperature precisely
In terms of production benefits, those advantages adds up to the following:
No contamination risk. Because infrared sensing devices can be placed well outside of the production environment, there is no risk of contaminating the production environment or what is being produced.
Flexibility and efficiency. Due to high response speeds and accuracy, infrared temperature-sensing devices are able to measure every object or volume of material in production while it is in motion. There is no need to temporarily stop a process and wait for a temperature reading of samples, as may be required with traditional thermocouple sensors.
Survivability and maintenance. Because infrared sensors do not need to be placed directly within the production environment, they can survive the monitoring of items at extremely high temperatures. In fact, many companies offer infrared devices that can measure temperatures beyond 6,000°F. They will also be safe from exposure to extremely harsh environments (those that are steamy, smoky or hot) where other sensors will be quickly destroyed and/or require regular replacement or maintenance intervals. With thousands of options available on the market today, and each offering unique benefits and advantages, selecting the right infrared temperature sensing method can be a complex process.
This article is intended to serve as a tutorial about the general categories of infrared temperature sensing technologies available, along with key consideration and factors in determining what is appropriate for your situation. It is meant to help you identify your requirements and needs before you begin your search.
IR SENSOR TECHNOLOGIES
Infrared temperature sensors typically consist of four basic components:
- A lens, which may vary depending on target-proximity variables
- An infrared energy detector
- Processing circuitry to convert the wavelengths into usable signals
- Input and output (I/O) capabilities, which can range from simple temperature reporting to complex visualization (such as 3-D thermal imaging), and/or alarm and control signals for external systems interoperability
Three general categories of infrared sensors are available:
Point — Spot thermometers. Measures temperature of a small surface point or area.
Line — Scanning thermometers. Measures temperature points along bands of space as the sensor scans back and forth across the target surface.
Area — Thermal imaging systems. Measures temperature covering an area as a grid, captured either as still images or motion video, with each cell or pixel representing a temperature.
There are literally thousands of options available on the market today, each with their own advantages and capabilities. With so many choices, the best place to begin is in identifying the application requirements.
Spot thermometers
Spot thermometers (also referred to as pyrometers) are ideal for situations where you need to continually measure a small area. They are also highly suitable when you need only a narrow set of features to get the desired results, and maintaining low cost is a necessity. These devices typically read only a narrow band of infrared wavelengths, which in turn can increase accuracy at that particular wavelength.
The ideal measurement area can be precisely small (starting at around 0.1 in. dia.) or wider, depending on lens choice and distance to target, with the temperature of the whole spot area averaged to produce output as a single temperature.
If you need to continuously measure large surface areas with different measurement points, a good strategy is to install multiple spot thermometers near each other and aim them at different target areas.
The output of spot thermometers typically consists of a single temperature (an average of the spot area being targeted), as well as an appropriate output signal output for interfacing with external systems.
Scanning thermometers
Scanning thermometers typically incorporate a single sensing unit with optical movement (such as a spinning mirror to redirect the beam) to continuously scan and measure surface temperatures. A scanning thermometer can serve a similar function to that of a multiple spot sensor for covering a wide area, while having lower overall cost and space requirements and more feature-rich output.
Infrared scanners are typically bundled with complex computer processing and software that is designed to aid in configuring the scanning area and enhance visualization of what has been scanned. More-advanced systems are capable of generating 3-D thermal imagery and providing complex control outputs to operate with external systems.
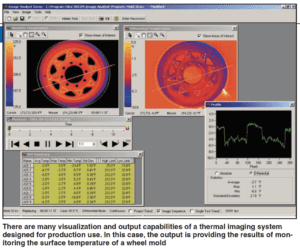
There are many visualization and output capabilities of a thermal imaging system
designed for production use. In this case, the output is providing the results of monitoring
the surface temperature of a wheel mold
Thermal imaging systems
A thermal imaging solution is like having 1,000 spot sensors monitoring 1,000 points of a target area all at once — with each pixel of the resulting image representing a temperature. In addition to generating an image, in most cases, data about the temperature of each pixel can be captured and stored for trending or I/O purposes.
Thermal imaging applications typically incorporate complex computer processing, and are used with inspection applications where there is a need to monitor an area (small or large) in finite detail.
This method can be useful in a production situation where a very rapid inspection solution is needed, and the rate of product heating or cooling is fast. In such cases, if any cluster of pixels within a defined area of a product’s thermal image fall above or below a prescribed temperature, the system can automatically initiate an action of some sort, such as diverting or tagging that product for a reject pile or some other corrective measure — all done in milliseconds.
A few key variables to consider in the choice between IR temperature measurement methods include temperature decay rate, process speed, and response time — or simply, how rapidly objects being measured cool down and/or move past the sensor, and how fast the sensing method can detect the amount of area needing to be covered, convert it all to temperature data and initiate the necessary outputs. Granted, infrared energy can be detected at the speed of light; but, processing limitations between the types of detecting equipment will vary depending on the particular situation.
For example, both infrared line scanners and infrared thermal imagers offer advantages with certain applications. Whereas scanners may take a few seconds to scan back and forth to generate a picture of an area, a thermal imaging system can monitor the same area all at once, though not always as accurately. In other words, in an application where the temperature decay rate is extremely rapid and you need to measure a whole object’s surface area at once, a thermal imaging solution is probably your best choice. On the other hand, if the target material is continuous and moves along a conveyor rapidly (such as glass sheets or piles of dry chemicals), higher temperature precision in necessary, and temperature decay is not rapid. In this case, an infrared line-scanning system or series of spot thermometers placed a strategic points may be more appropriate.
IDENTIFYING YOUR NEEDS
General considerations
Taking the time to outline and prioritize your requirements will aid you in your search. As you investigate the offerings of multiple vendors, refine your scope as you eliminate the options that do not fit. The remainder of this article is meant to raise questions that you may need to consider, in order to help you prioritize your needs.
Most vendors will guide you in identifying the particular products to suit your requirements. Many will offer their expertise to help tailor a system (including software and hardware custom-tailoring — for a fee, of course) to meet your requirements. To do so, they will begin with an investigation of your intended applications for the sensor, and the unique circumstances of your situation. Typical initial open-ended questions might include:
- What specifically are you trying to measure, and why is it important?
- What is the chemical makeup of the material you are trying to measure?
- How fast is the process or target moving?
- Are there particular properties of the material being measured that need to be considered? For example, does the material or object change state or color during heating and cooling-down processes? Does the material or object have a surface coating that may affect the temperature readings of what you are trying to measure?
- What are some of the production challenges (small viewing areas, windows or solid obstructions, high-temperature and smoky or steamy environments, fast processing time) that you are facing in the area that you are needing to measure?
- What sorts of outputs and benefit outcomes are you expecting from a solution?
These questions are designed to draw generalizations about the challenges you face and the goals you seek to attain through the system so that the vendor can guide you appropriately.
With the answers to those or similar questions in hand, the next step is to consider how your application needs fit in the context of the infrared temperature sensors that exist.
Choosing among IR options
Outlining responses to these questions will be key to helping determine which infrared sensing technology will best serve your needs.
- What are the dimensions of the target area(s) you are trying to measure?
- Will an average temperature of that area do, or do you need more finite measurement?
- Is the target area flat or dimensional? Does the surface have a texture to be factored?
- How rapidly is the target moving or cooling/warming in the area you want to measure?
- What types of system outputs are you looking to achieve?
- Will spot temperature average, and display of a single temperature do the job?
- Do you need comprehensive data recording, reporting and/or imaging of an object or area?
- Do you need alarm outputs and/or control outputs (to equipment both upstream and downstream of the measurement area)?
Temperature span
The temperature-range specifications of the sensor will likely be the first and most basic criterion that you will assess in eliminating one offering versus another. If a technology’s upper and lower measurements limits are not greater than the temperature range that needs to be measured, then it will likely not meet your needs.
Degree of portability
The needed degree of portability of the equipment will depend on your intended applications. Typically, a dedicated-purpose sensor is one that will sit in a fixed position in a process for many years to serve a rigid and narrow function. In such cases, the unit is designed for limited portability, if any at all. Also, because it is meant to serve a dedicated purpose, the spectral and temperature range and many other features tend to be limited, as additional features are unnecessary. Finally, the casings for fixed or dedicated-purpose sensors are designed to be highly durable to withstand long periods within extremely high ambient temperatures, or smoky and steamy environments.
Portable units, on the other hand, tend be designed for lightweight, handheld use, and are more broadly featured in order to accommodate a variety of temperature sensing situations and materials. Because of the broad and flexible application range, portable units also tend to be more expensive and less precise (if measuring wide areas and ranges of materials).
Target emissivity and sensor spectral range
Probably the most difficult concept to understand, and the area that will require the most assistance, is that of determining the target material’s emissivity. A target’s emissivity ranking, and relation to the appropriate sensor spectral range are important to assuring accurate temperature readings.
Emissivity is a measure of an object’s surface emittance, or put more simply — a measure of a material’s ability to absorb and radiate energy. A material’s emissivity ranking is presented as a number between 0 and 1, and represents the factor of a particular material’s emittance as compared to a perfect emitter — a blackbody source.
An infrared sensor’s spectral or wavelength range (indicated in terms of microns) refers to the span(s) measured by the sensor within the infrared spectrum. The shortest wavelength utilized is 0.65 microns and the longest is 8–14 microns. Here are some guidelines regarding typical applications at the various wavelengths:
- As wavelength increases, lower temperatures can be measured. For instance, at 0.65 microns, the lowest temperature that can be measured is 1,400°F (700°C). Compare that to the 8–14 micron range, where the lowest measurable temperature is –50°F (–50°C)
- Wavelengths of 0.65 to 2.6 microns are used for metals, and can see through quartz windows. Meanwhile, they are available with fiber optic technology
- Wavelengths of 3.4 and 7.9 microns are used to measure thin plastic films. At these wavelengths, films as thin as 0.001 are opaque and only the surface temperature is measured
- The wavelength of 5 microns is used to measure glass windows and containers
- The 7.9-micron wavelength is utilized for ceramics and very thin glass
- The 8-14 micron wavelength is commonly used to measure low temperatures of textiles, paper and food. It is a very common wavelength for portable infrared thermometers
Generally, portable devices are designed to support wide bandwidth ranges (to ensure compatibility with a wide range of sensing situations) whereas sensors designed for fixed, inline use tend to support narrow bandwidth ranges (to ensure high accuracy at reading temperature of particular material). In the latter case, reading a narrow bandwidth helps eliminate readings of other materials or environmental factors (such as water or gas vapors or surface reflections) that may interfere with readings of a particular target material.
In most cases, the sensor provider will offer to test and determine the emissivity ratio of sample material that you send, and to guide you in determining the appropriate sensor bandwidth for your situation. Emissivity and wavelength charts for common materials can be found on the websites of most infrared sensor manufacturers or by using one of the major searching engines.
Environmental challenges and necessary accessories
The environment within which the sensor will be used, particularly a fixed position unit, needs to be assessed. Beyond the sensing capabilities internal to the unit, external factors incorporated in the overall sensor design need to be taken into account:
- Is the sensor mounting or viewing area limited?
- Are there physical obstructions, as well as process-related obstructions, to consider (such as smoke, dust, steam or gases) in the viewing area to the target?
- Will the sensing unit be subjected to, and be able to withstand years of exposure to, high temperatures, smoke, dust or steam?
- Could a high ambient temperature of the process further rise and thus throw off the accuracy of the sensor?
Outlining the environmental factors you face will help you in determining the system capabilities or any ancillary accessories you might need to factor to ensure long term sensor protection, accuracy and reliability.
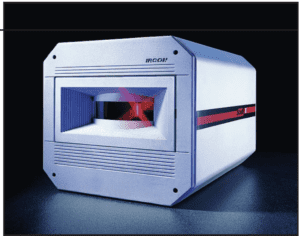
Infrared temperature scanning units continuously measure surface temperatures
Fiber-optic lens attachment. Most spot infrared thermometer manufacturers offer the option of a small lens housing that attaches through a fiber optic cable to the sensor case. This allow the lens to be mounted in a small or enclosed area to point at a hard-to-reach target, while placing the actual sensing electronics at a safer distance outside of the production environment.
Air purge and/or dirty window detector. An air purge, attached to a compressed air source and placed over the sensor lens, is designed to blow a constant stream of air across the sensor window or lens, thereby preventing the collection of dust, vapor or smoke particles. Meanwhile, a dirty window detector is a small specialized sensor designed to monitor the sensor lens for particulates — if too much material collects, it will send an alarm. Both options assure greater accuracy and lower maintenance time and hazards, reducing the need to continuously clean the lens of a sensor that is placed in hard to reach or dangerous locations.
Air or water jackets. Jackets are designed to encase and protect a sensor from high ambient temperatures and other environmental challenges. Circulating air or water through the lining of the jacket helps better assure that the sensor is operating within a range that will not destroy the sensor or affect accuracy of its temperature readings.
Authors
Robert Janecek is a marketing specialist for IRCON, Inc. (7300 N Natchez Ave., Niles, IL 60714; Phone: 847-967-5151 x259; Fax: 847-647- 0070; Email: [email protected]), a company that specializes at designing, manufacturing and selling non-contact infrared sensing technologies for production applications. Founded in 1962, Ircon offers extensive expertise and a comprehensive line of instruments, software and support services that can be tailored to assist a wide range of manufacturing process monitoring needs. Headquartered in Niles, the firm offers products that are sold worldwide, through a network of nearly 150 distributors. Robert has 17 years experience managing business-to-business marketing and communications programs (including prior roles at Ameritech Information Systems and Siemens Building Technologies), specializing in marketing automation solutions, and experience targeting a wide range of industries. Robert holds a B.S. in commercial graphics from Southern Illinois University and an M.Sc. in managerial communications from Northwestern University, and is currently working to complete an M.B.A. at Lake Forest Graduate School of Management.
Vern Lappe is vice president of technical services for IRCON, Inc. (Phone: 847-967-5151; Email: [email protected]), He has worked for IRCON, Inc for over 30 years serving in a variety of leadership roles, and serving customers from a wide range of industries. His technical aptitude and application experience regarding infrared temperature measurement are unparalleled, and he is regarded as a leading expert in this field. Lappe possesses a B.S.M.E. degree, with a focus on heat transfer.