Sponsored by
Silos and bins fail with a frequency which is much higher than almost any other industrial equipment. Sometimes the failure only involves distortion or deformation which, while unsightly, does not pose a safety or operational hazard. In other cases, failure involves complete collapse of the structure with accompanying loss of use and even loss of life.
Presented are numerous case histories involving structural failure which illustrate common mistakes as well as limits of design.
1. INTRODUCTION
Although statistics are not available, hundreds of industrial and farm silos, bins, and hoppers experience some degree of failure each year. [1-3] Sometimes the failure is a complete and dramatic structural collapse. Other times the failure is not as dramatic or as obvious. For example, cracks may form in a concrete wall, or dents in a steel shell, either of which might appear harmless to the casual observer. Nevertheless, these are danger signals which indicate that corrective measures are probably required.
The economic cost of a silo failure is never small. The owner faces the immediate costs of lost production and repairs, personnel in the vicinity are exposed to significant danger, and the designer and builder face possible litigation because of their liability exposure.
The major causes of silo failures are due to shortcomings in one or more of four categories: design, construction, usage, and maintenance. Each of these is explored below, with examples and lessons learned.
2. FAILURES DUE TO DESIGN ERRORS
Silo design requires specialized knowledge. The designer must first establish the material’s flow properties [4], then consider such items as flow channel geometry, flow and static pressure development, and dynamic effects. Problems such as ratholing and self-induced silo vibration have to be prevented, while assuring reliable discharge at the required rate. Non-uniform loads, thermal loads, and the effects of non-standard fabrication details must be considered. Above all, the designer must know when to be cautious in the face of incomplete or misleading information, or recommendations that come from handbooks, or from people with the “it’s always been done this way” syndrome.
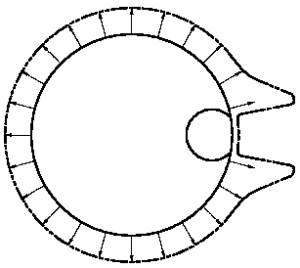
Fig. 1, Non-uniform pressures caused by eccentric withdrawal
Having established the design criteria, a competent design has to follow. Here the designer must have a full appreciation of load combinations, load paths, primary and secondary effects on structural elements, and the relative flexibility of the elements. [5,6] Special attention must be given to how the most critical details in the structure will be constructed so that the full requirements and intent of the design will be realized.
Five of the most common problems which designers often ignore are described below, along with a few examples of each.
2.1 Bending of circular walls caused by eccentric withdrawal
This is one of the most common causes of silo structural problems, since it is so often overlooked. It results when the withdrawal point from the hopper is not located on the vertical centerline of a circular silo [7,8], and is particularly common when using silos with multiple hoppers in which only one or two of the hopper outlets are used at a time. If the resulting flow channel intersects the silo wall, non-uniform pressures will develop around the circumference of the silo leading to horizontal and vertical bending moments. See Figure 1. Many silo designers incorrectly account for these non-uniform pressures by only increasing hoop tension. [9,10]
Some examples:
- A silo storing sodium sulfate consisted of a 4.3 m diameter by 15 m tall cylinder section, below which was a short conical hopper, a transition hopper, and 460 mm diameter screw feeder. A significant inward dent developed about mid-height in the cylinder section. It extended about one-quarter of the way around the circumference and was centered slightly offset from the long axis of the screw at its back end. The problem was caused by eccentric withdrawal due to an improperly designed screw feeder. See Figure 2.
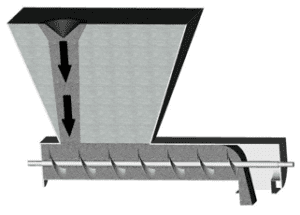
Fig. 2, Constant pitch screw feeder caused eccentric withdrawal
- A silo consisting of a 3.5 m diameter cylinder, 20° (from vertical) cone section, 3 m diameter vibrating discharger, and pantleg discharge chute was used to store reground PVC flake. Flow was metered through each chute leg using a rotary valve. The vibrating discharger was used infrequently (30 sec. on, 5 minutes off), and only one leg of the pantleg was used most of the time. A dent formed in the cylinder section centered over the active pantleg.
A blending silo utilized 24 external tubes to withdraw plastic pellets at various elevations from the cylinder and cone sections. Significant wrinkles developed in the cylinder section above several of the tubes. The lessons to be learned here are:
- Whenever possible, design your silo for center fill and center withdrawal.
- If eccentric fill or withdrawal is contemplated, perform a structural check first to make sure that the silo can withstand the non-uniform loading conditions and resulting bending moments.
- Be particularly careful with silos that have an elongated hopper outlet. An improperly designed screw feeder or belt feeder interface, or a partially opened slide gate, will often result in an eccentric flow pattern with accompanying non-uniform loads.
- If a sweep arm unloader is used, be aware that operating it like a windshield wiper (back-and-forth in one area) will create a preferential flow channel on one side of a silo.
- If multiple outlets are required, consider splitting the discharge stream outside of the silo below the main central withdrawal point.
- If a vibrating discharger is used but not cycled on and off on a regular basis, an eccentric flow channel may form, particularly if a pantleg chute is below the outlet.
- Consider non-uniform pressures when designing silos with blend tubes.
2.2 Large and/or non-symmetric pressures caused by inserts
Support beams, inverted cones, blend tubes, and other types of internals can impose large concentrated loads and/or non-symmetric pressures on a silo wall leading to unacceptable bending stresses.
Two examples:
- A tear developed in the cone section of a 4 m diameter silo storing reground polyester pellets. This tear was located where a support strut for an inverted conical insert was welded to the cone wall. Upon emptying the silo, it was found that the insert support plates were severely deformed and detached from the cone wall.
- Tests showed that a certain agglomerate could experience particle attrition under the loads generated in a large silo. To reduce the potential of this happening, an insert was designed to be located in the cylinder section of an 8 m diameter silo. This 15 m tall inverted cone extended from just below the transition to within 2 m of the top of the silo. The designers were provided with the loads, which would act on this insert; however, they believed the values to be too conservative, so they designed the support structure for smaller loads. Shortly after being put into operation, the insert supports failed, causing the insert to fall and impact a BINSERTÒ inner cone below, the supports of which also failed as a result of the impact.
Lessons learned:
- Don’t ignore loads on inserts, since they can be extremely large. [11] In addition, non-uniform pressures may develop if the flow pattern around the insert is even slightly asymmetric.
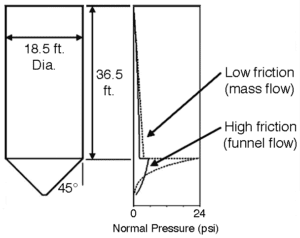
Fig. 3, Comparison of wall normal pressures due to assumed funnel flow and actual mass flow
- Open inserts (such as a BINSERTÒ or blend tube) can also have large loads acting on them. Consideration must be given to the consequences of the insert becoming plugged, thereby preventing material from flowing through it. In this case, the vertical load greatly exceeds the dead weight of the material inside the insert and the cone of material above it.
2.3 Ignoring flow patterns and material properties
Sometimes mass flow develops in silos, which were structurally designed for funnel flow. [4] Even if this doesn’t occur, the local pressure peak, which develops where a funnel flow channel intersects a silo wall, can be devastating. [6]
In some circumstances, ignoring the properties of the bulk solid to be stored can be worse than assuming an incorrect flow pattern. Consider, for example, designing a steel silo to store coal. Lacking a sample of coal which could be tested to form the design basis, the designer may resort to an often quoted design code [12] which lists the wall friction angle for “coal on steel,” with no consideration as to the type of coal, its moisture, particle size, ash content, or the type of steel, its surface finish, etc. Flow and structural problems are common when this approach to design is taken.
Two examples:
- Several bolted silos storing lubricated plastic pellets split apart along a radial seam near the top of the hopper section. Although the silos were designed structurally for funnel flow, no flow tests were performed to see if this flow pattern would occur. Lab tests performed after the failure showed that mass flow developed along the 45° cone walls. See Figure 3.
- Two similar bolted silos also storing plastic pellets failed in a similar manner. Lab tests showed that the wall friction was not low enough for mass flow. However, the wall friction angle was much lower than the silo designer assumed. Thus, less of the pellet mass was supported by shear along the vertical cylinder walls, resulting in much higher wall pressures in the hopper than was assumed by the designer. See Figure 4.
Lessons learned:
- Know your material’s flow properties, and the type of flow pattern that is likely to develop in your silo. [13]
- If the flow properties are likely to vary (due, for example, to changes in moisture, particle size, temperature, different suppliers), make sure that the silo is designed to handle this variation.
- If your design is close to the mass flow/funnel flow limit, consider the possible effects of slight changes in material properties or the interior surface of the silo (particularly its hopper section). The latter is particularly important if the hopper walls are likely to be polished with use.
- Buyers beware! If you don’t know which flow pattern is going to develop in your silo, or the possible consequences of designing for the wrong one, retain the services of a silo expert who can advise you.
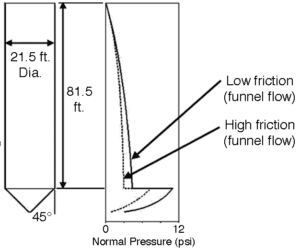
Fig. 4, Comparison of wall normal pressures due to assumed high wall friction and actual low friction
- Using tables of values of material properties is risky at best and should only be used as a last resort if no samples of the actual material to be stored are available. A better approach would be to check with a silo expert who may have past experience handling the material. Inclusion of additional safety factors in the design, to account for unknown variations, is also often warranted.
2.4 Special considerations with bolted tanks and reinforced concrete construction
Many silos are constructed of bolted metal panels (usually steel or aluminum), while others are constructed of reinforced concrete. Both types of construction have specific design requirements.
Bolted connections transfer loads through various load paths, and can fail in at least four different modes: bolt shear, net section tension, hole tear-out, and piling around bolt holes. Which mode results in the lowest failure load depends on specifics of the metal (e.g., its yield and ultimate strengths, thickness), the bolts (e.g., size, strength, whether or not fully threaded, how highly torqued), spacing between bolt holes, number of rows of bolts, etc. [14-16]
Compressive buckling must also be considered, particularly if the bolted silo has corrugated walls or is constructed from aluminum.
Reinforced concrete construction presents different problems [17,18]. Concrete is strong in compression but very weak in tension. Thus, reinforcing steel is used to provide resistance to tensile stresses. A silo that has only a single layer of horizontal reinforcing steel is capable of resisting hoop tension, but has very little bending resistance; therefore if non-uniform pressures occur (e.g., due to an eccentric flow channel), the silo is likely to crack. Unfortunately, the inside face of the silo wall, where cracks are difficult to detect, is where the maximum tensile stresses due to bending are most likely to occur. Undetected cracks can continue to grow until the silo is in danger of imminent collapse.
An example:
- Vertical cracking of concrete was observed in a 21 m diameter raw coal silo shortly after it was put into operation. The cracks were located in the portion of the silo that contained a single layer of reinforcing steel. In an attempt to stop the cracks from growing further, they were injected with an epoxy, but this proved ineffective. Later, post-tensioning strands were added to the outside of the silo. Five years later, enough delamination had occurred on the inside of the wall to expose significant lengths of rebar and allow them to be pulled out and drop down the wall. Extensive repairs and reinforcing were required in order for the silo to be used safely.
Lessons learned:
- Consider all the various modes by which a bolted joint can fail, and follow recognized design procedures.
- Check to ensure that the design can withstand compressive buckling.
- Determine the likelihood of eccentric fill or discharge and design accordingly. In particular, do not use a single layer of reinforcement if eccentric loading is possible.
2.5 Special considerations concerning temperature and moisture
The walls of outdoor metal silos can expand during the day and contract at night as the temperature drops. If there is no discharge taking place and the material inside the silo is free flowing, it will settle as the silo expands. However, it cannot be pushed back up when the silo walls contract, so it resists the contraction, which in turn causes increased tensile stresses in the wall. This phenomenon, which is repeated each day the material sits at rest, is called thermal ratcheting. [19-23]
Another unusual loading condition can occur when moisture migrates between stagnant particles, or masses of stagnant particles, which expand when moisture is added to them. If this occurs while material is not being withdrawn, upward expansion is greatly restrained. Therefore, most of the expansion must occur in the horizontal plane, which will result in significantly increased lateral pressures on, and hoop stresses in, the silo walls.
Two examples:
- A 24 m diameter bolted steel silo storing fly ash split apart about two weeks after it was first filled to capacity. Nearly 10,000 tons of fly ash discharged in the accident, which occurred at night when no fly ash was being filled into or discharged from this silo. Calculations revealed that the silo was underdesigned, and the probable cause of failure was thermal ratcheting.
- A 7.3 m diameter silo stored a mixture of wet, spent brewer’s grains, corn, and other ingredients. No problems occurred as long as the material was not stored for any significant time. However, after sitting several days without discharge during a holiday period, the silo walls split apart dropping 700 tons of material onto the ground. Strain gauge tests in a lab test rig showed that when moisture migration caused the corn particles to swell, pressures on the silo wall increased by more than a factor of five.
Lessons learned:
- Include factors of safety in the design of outdoor metal silos to account for the effects of thermal ratcheting. [24]
- Assess the likelihood of significant moisture migration occurring while the bulk solid is stationary, and design accordingly.
3. FAILURES DUE TO CONSTRUCTION ERRORS
In the construction phase, there are two ways in which problems can be created. The more common of these is poor workmanship. Faulty construction, such as using the wrong materials or not using adequate reinforcement, and uneven foundation settlement are but two examples of such a problem.
The other cause of construction problems is the introduction of badly chosen, or even unauthorized, changes during construction in order to expedite the work or reduce costs.
3.1 Incorrect material
Close inspection of contractors’ work is important in order to ensure that design specifications are being followed. This includes checking for use of correct bolts (size, strength, etc.), correct size and spacing of rebar, specified type and thickness of silo walls, etc.
An example:
- During investigation of the fly ash silo failure described above (2.5), it was discovered that less than 1% of the bolts recovered had the specified marking on their head, and none of these were used in the critical vertical seams. Strength tests on these incorrect bolts revealed that some had tensile strengths less than the minimum required for the specified bolts.
Lessons learned:
- Use only qualified suppliers and contractors.
- Closely inspect the installation.
- Make sure that specifications are clear and tightly written [25].
3.2 Uneven foundation settlement
Foundation design for silos is not appreciably different than for other structures. As a result, uneven settlement is rare. However, when it does occur, the consequences can be catastrophic since usually the center of gravity of the mass is well above the ground.
Example:
- A 49 m diameter by 14.5 m tall grain silo experienced a catastrophic failure one cold winter night. Investigation revealed that because of inadequate design of the concrete footing and changes to it during construction, the foundation was significantly weakened. Failure occurred when the contents of the silo exerted outward forces on the steel shell, which overloaded the foundation causing it to crack. The failing foundation in turn pulled out on the steel shell. Low temperatures created additional thermal stresses at the bottom of the shell.
Lessons learned:
- Use experienced soils engineers and foundation designers.
- Use reputable contractors.
- Closely inspect the work. (See comments above in Section 3.1.)
3.3 Design changes during construction
Unauthorized changes during construction can put a silo structure at risk. Seemingly minor details are often important in ensuring a particular type of flow pattern (especially mass flow), or in allowing the structure to resist the applied loads.
Example:
- A buckle was observed in the side wall of a spiral aluminum silo storing plastic pellets. Once the silo was emptied it was discovered that many of the internal stiffeners had also buckled in the region of the shell buckling. Analysis revealed that the most probable cause of buckling was lack of sufficient welds between the stiffeners and the shell.
Lessons learned:
- Make sure that both the silo builder and designer carefully consider and approve any changes in details, material specifications, or erection procedure.
- Closely inspect all construction.
4. FAILURES DUE TO USAGE
A properly designed and properly constructed silo should have a long life. Unfortunately, this is not always the case. Problems can arise when the flow properties of the material change, the structure changes because of wear, or an explosive condition arises.
If a different bulk material is placed in a silo than the one for which the silo was designed, obstructions such as arches and ratholes may form, and the flow pattern and loads may be completely different than expected. The load distribution can also be radically changed if alterations to the outlet geometry are made, if a side outlet is put in a center discharge silo, or if a flow-controlling insert or constriction is added. The designer or a silo expert should be consulted regarding the effects of such changes before they are implemented.
4.1 Dynamic loads due to collapsing arches or ratholes, self-induced vibrations, or explosions
When a poorly flowing material is placed in a silo which was not designed to store and handle it, flow stoppages due to arching or ratholing are likely. Sometimes these obstructions will clear by themselves, but, more often, operators will have to resort to various (sometimes drastic) means to clear them. No matter which method is used, the resulting dynamic loads when an arch or rathole fails can collapse the silo. [26]
Self-induced silo vibrations can also result in significant dynamic loads for which most silos are not designed to withstand. [27,28] In addition, few if any silos can withstand the loads imposed by an explosion — either internal or external.
Two examples:
- A 13 m diameter by 23 m tall reinforced concrete silo stored waste coal. Below the cylinder was a 30° conical hopper terminating at a 4.6 m diameter vibrating discharger. Flow from the silo was controlled by a vibrating pan feeder. A rathole formed above the discharger, then partially collapsed. The resulting impact separated the vibrating discharger from the cone section and drove the vibrating pan feeder into the floor.
- Three large bolted steel silos were used to store distiller’s dry grain with solubles. Each silo’s cylinder section was 7.9 m in diameter by 15 m tall, below which was a 30° conical hopper and 3 m diameter vibrating discharger. Flow was controlled with a 300 mm diameter screw feeder. Severe structural damage occurred in all three silos, including 300 to 900 mm indentations in portions of the cylinder walls, two completely split radial seams in one of the static hopper sections, and one of the vibrating dischargers dropping off from its supports. The structural problems were directly related to the poor flow characteristics of the material. In fact, its flow properties were so poor that plant personnel occasionally resorted to using dynamite to break it up!
Lessons learned:
- Know the flow properties of your material and the flow properties assumed in the design of your silo. If the source of your material changes, or if you plan to store a different material in your silo, have the new material tested for flow properties. Get advice from experts before putting the new or changed material into your silo.
- Use extreme caution in attempting to restore flow if an arch or rathole forms. Under these circumstances, personnel should not be allowed to be in close proximity to the silo. Consideration should be given to top reclaim using experts trained in this technique.
- Avoid accumulations of dust or ignitable gases, which could cause an explosion.
4.2 Changes in flow patterns
Changing material properties or polishing of the inside surface of the silo may cause mass flow to develop in a silo which was structurally designed for funnel flow. (The opposite can also occur – funnel flow in a silo designed structurally for mass flow – but this generally is not as serious a problem.) Mass flow will result in a dramatically different wall pressure loading than with funnel flow, particularly at the top of the hopper section.
Two examples:
- Six 7.9 m diameter by 22 m. tall silos were used to store high-density polyethylene fluff and pellets. Below each cylinder section was a 30° cone terminating at a rotary valve feeder. A radial hopper seam split open on one silo, spilling one million pounds of material onto the ground. The cause of this failure was determined to be mass flow loads. The silo was structurally designed only for funnel flow. See Figure 5.
- Four outdoor bolted silos were used to store barley and corn. As with the previous example, failure occurred by splitting of a radial seam near the top of the hopper, which was the result of unexpected mass flow loads. In this case, the cone walls were apparently polished by the barley, and the wall friction decreased further when the outside air temperature dropped below freezing.
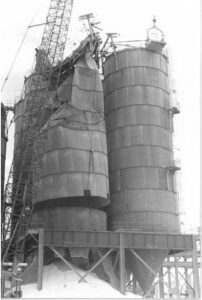
Fig. 5, End-result of mass flow developing in a silo designed structurally for funnel flow
Lessons learned:
- Know your material’s flow properties and the flow properties used in the design. Avoid materials and/or conditions that could result in a flow pattern for which the silo was not designed.
- Routinely inspect the interior of your silo, checking for abrasion marks, which may indicate mass flow. [29]
- Inspect the exterior of a bolted silo on a regular basis. Pay particular attention to the bolted joints near the top of the hopper, noting any waviness along the edges of the sheets, elongation of bolt holes, or cracks between bolt holes, all of which are signs of over-stress.
4.3 Buckling of unsupported wall
A pressurized cylinder is more resistant to compressive buckling than an unpressurized one. [9] In addition, if this pressure is caused by a bulk solid (as opposed to a liquid or gas), it is even more resistant. The reason is as follows: Gas or liquid pressure is constant around a silo’s circumference and remains unchanged as the silo starts to deform. On the other hand, the pressure exerted by a bulk solid against a silo’s wall increases in areas where the walls are deforming inward, and decreases where the walls are expanding. This provides a significant restraining effect once buckling begins.
Now consider what happens if an arch forms across a silo’s cylinder section, and material below it is withdrawn. Not only is the restraining effect of the bulk solid lost, but the full weight of the silo contents above the arch are transferred to the now unsupported region of the silo walls. Buckling failure is likely when this occurs.
Example:
- A 7.6 m diameter by 27 m tall bolted flat-bottom silo was used to store soybean meal. Discharge occurred by a sweep arm screw unloader. The material’s flow properties varied considerably, from free flowing to extremely cohesive. An arch formed above the unloader, and spanned the full diameter of the silo. Material below this was removed by the unloader, so the full one million pounds was transferred to the unsupported thin silo wall causing it to fail by vertical buckling. See Figure 6.
Lessons learned:
- Know your material’s flow properties.
- If flow stops, investigate the cause before attempting to restart discharge.
5. FAILURES DUE TO IMPROPER MAINTENANCE
Maintenance of a silo comes in the owner’s or user’s domain, and must not be neglected. Two types of maintenance work are required. The first is the regular preventative work, such as the periodic inspection and repair of the walls and/or liner used to promote flow, protect the structure, or both. Loss of a liner may be unavoidable with an abrasive or corrosive product, yet maintaining a liner in proper working condition is necessary if the silo is to operate as designed. Other examples of preventative maintenance items include roof vents, level probes, feeders, dischargers, and gates.
The second area of maintenance involves looking for signs of distress (e.g., cracks, wall distortion, tilting of the structure) and reacting to them. [29] If evidence of a problem appears, expert help should be immediately summoned. An inappropriate response to a sign that something is going wrong, including the common instinct to lower the silo fill level, can cause a failure to occur with greater speed and perhaps greater severity.
5.1 Corrosion and erosion
Silo walls thinned by corrosion or erosion are less able to resist applied loads than when they were new. This is a particular problem when handling abrasive materials or when using carbon steel construction in moist or otherwise corrosive environments. Combining the effects of abrasion with corrosion significantly accelerates the problem. This can occur, for example, with special aging steels. Abrasive wear causes the surface layer to be removed, thereby exposing new material and speeding up the aging process which significantly weakens the structure.
Three examples:
- A coal silo was fabricated from aging steel. After about five years of use, the hopper detached from the cylinder section while the silo was full. The cause was determined to be thinning of the silo wall due to abrasion from coal and corrosion.
- A tile silo storing coal failed after many years in use. This progressive failure occurred because of weathering effects on the exterior and corrosive conditions due to wet coal on the interior. These combined to corrode the steel reinforcing bars, which then failed.
- Six coal silos at a chemical plant lasted for about 30 years, after which time two of the six experienced a structural failure, which prompted a close inspection of all six silos. The carbon steel walls were found to have thinned significantly, to the point that actual holes were visible in places. Corrosion, both exterior and interior, was to blame.
Lessons learned:
- Carefully inspect your silos on a regular basis. Determine the minimum wall thickness required for structural integrity and compare to the actual wall thickness.
- Do not use aging steels for silo construction if the surface will be exposed to abrasive wear.
- Prevent buildup of material, which could trap moisture on the exterior of outdoor silos.
5.2 Lack of routine inspection
Silo failures often cause significant damage and sometimes result in death. Often these failures could have been prevented or the damage could have been minimized with information that could have been gained through routine inspection.
Example:
- The hopper section of a stone bin at a mining operation fell off when the bin was full, killing a person working below. The problem was particularly attributed to material buildup on horizontal external structural members which, combined with moisture from the air, created a corrosive environment, resulting in excessive thinning of the silo wall.
Lessons learned:
- Inspect silos routinely, both internally and externally. [29] This is particularly important with bolted and reinforced concrete silos, and silos which are exposed to a corrosive environment. For example, look for any signs of corrosion, exposed rebar, unusual cracking, or spalling of concrete.
- If conditions change (e.g., a different material is to be stored) or unusual events occur (e.g., very high winds, an earthquake), inspect the silo before putting it back in operation.
- Perform a detailed structural inspection before designing modifications to a silo.
5.3 Improper reaction to signs of distress
A common reaction to signs of silo distress is to ignore them, often because personnel are unaware of both the meaning and consequences of doing so. Another common reaction is curiosity. People have lost lives because, due to their curiosity, they were in the wrong place at the wrong time. Even if danger signs are understood, it is common for inappropriate action to be taken in an attempt to “reduce” the chance of failure. In some extreme cases, catastrophic failure has been induced where, with appropriate action, the damage could have been relatively minor.
Two examples:
- A bolted steel silo with a sweep arm unloader was used to store soybean meal. The meal hardened, so the sweep arm was operated back and forth to try to discharge the meal. This process continued for some time, even though wrinkles were observed in the silo wall above the area where the sweep arm was operating. Eventually the indentations became so great that the silo collapsed.
- Another bolted silo storing grain stood up some 14 years before failure. Shortly after startup in the spring after the grain had been sitting essentially stationary all winter, the silo started tilting at approximately mid-height. Not realizing the consequences of continued withdrawal, the owner operated the discharge system. Two days later, the silo collapsed completely.
Lessons learned:
- Since a weakened silo is a very dangerous structure, limit access to the area surrounding it to only those personnel who need to be there, and make sure that they have the education and experience to deal with the situation. Extreme caution should always be exercised.
- At the first sign of silo distress, cease discharging immediately and assess the integrity of the structure.
- Investigate the cause of the distress. Retain experts with knowledge of silo structures to assist in the investigation.
6. CONCLUSIONS
Silos that are designed, built, operated, and maintained properly, will provide long life. Each of the case histories given above illustrates the effects of one or more of the shortcomings possible in design, construction, usage, and maintenance. In each example, the cost of repairs or rebuilding, the cost of litigation, and the cost of insurance added up to several times the cost of doing the job properly in the first place.
The best approach to the design of a silo, bin, or hopper for bulk materials is one that is reasoned, thorough, conservative, and based on measured parameters. Design engineers are not legally protected by sticking to a code of practice. Compliance with the locally applicable code is, of course, necessary, but it should never be regarded, by itself, as a sufficient condition to the performance of a satisfactory design.
It is the responsibility of the designer to ensure that the design is based on sound, complete knowledge of the materials being handled, that the design is competent, and that it covers all foreseeable loading combinations. It is the joint responsibility of the designer, builder, and owner that construction is of an acceptable standard, and fulfills the intent of the design. It is then the responsibility of the owner to properly maintain the structural and mechanical components. It is also the responsibility of the owner to ensure that any intended alteration in usage, discharge geometry or hardware, liner material, or any other specified parameter, is preceded by a design review with strengthening applied as required.
REFERENCES
- R. T. Jenkyn and D. J. Goodwill, Silo Failures: Lessons to be Learned, Engineering Digest, Sept. 1987.
- J. W. Carson and D. J. Goodwill, The Design of Large Coal Silos for Safety, Reliability and Economy, Bulk Solids Handling 4, pp. 173-177, 1984.
- J. Ravenet, Silos: Deformaciones – Fallas – Explosiones, Prevencion De Accidentes, Editores Técnicos Asociados, s.a.
- A. W. Jenike, Storage and Flow of Solids University of Utah Engineering Experiment Station, Bulletin No. 123, Nov. 1964.
- A. W. Jenike, Effect of Solids Flow Properties and Hopper Configuration on Silo Loads, In Unit and Bulk Materials Handling (Loeffler, F.J., and C.R. Proctor, eds.), ASME, 1980, pp 97-106.
- J. W. Carson and R. T. Jenkyn, Load Development and Structural Considerations in Silo Design, Presented at Reliable Flow of Particulate Solids II, Oslo, Norway, August 1993.
- A. W. Jenike, Denting of Circular Bins with Eccentric Drawpoints, Journal of the Structural Division, Proceedings of the American Society of Civil Engineers 93, pp. 27-35, 1967.
- T. Johnston, Analysis of Silo Failures from Asymmetric Flow, Presented at the 1991 Spring Convention, American Concrete Institute, Boston, MA, March 17-21, 1991.
- E. H. Gaylord and C.N. Gaylord, Design of steel bins for storage of bulk solids, Prentice-Hall, 1984.
- G. E. Blight, Defects in accepted methods of estimating design loadings for silos, Proc. Instn Cir. Engrs. 88 Part 1, pp. 1015-1036, Dec. 1990.
- J. R. Johanson and W.K. Kleysteuber, Structural Support of Flow-Corrective Inserts in Bins, Proceedings AIChE, Atlantic City, September 1966.
- ACI Standard 313-91, Standard practice for design and construction of concrete silos and stacking tubes for storing granular materials 1991.
- J. W. Carson and J. Marinelli, Characterize Bulk Solids to Ensure Smooth Flow, Chemical Engineering 101, no. 4, pp. 78-90, April 1994.
- AWWA Standard D103-87, Factory-coated bolted steel tanks for water storage 1987.
- Specification for the design of cold-formed steel structural members American Iron and Steel Institute, 1986.
- G. L. Kulak, J.W. Fischer and J.H.A. Struik, Guide to design criteria for bolted and riveted joints, 2nd ed. John Wiley, 1987.
- D. F. Themer, Failures of Reinforced Concrete Grain Silos, Transactions of the ASME Journal of Engineering for Industry, pp. 460 to 477, May 1969
- I.A.S.Z. Peschl, Construction of Concrete Silos, Silo Failures – An Analysis of the Reasons, Norwegian Society of Chartered Engineers, February 28-March 2, 1977
- G. E. Blight, Temperature changes affect pressures in steel bins, International Journal Bulk Solids Storage in Silos 1 No. 3 1985, pp. 1 to 7.
- G. E. Blight, Temperature surcharge pressures in reinforced concrete silos, Powder Handling and Processing 2 No. 4, November 1990, pp. 303 to 305.
- G. E. Blight, Measurements on full size silos part 1: temperatures and strains, Bulk Solids Handling 7, No. 6 December 1987 pp. 781 to 786.
- G. E. Blight, Temperature-induced loadings on silo walls, Structural Engineering Review 4 No. 1, 1992, pp. 61-71.
- H. B. Manbeck, Predicting thermally induced pressures in grain bins, Trans. ASAE 27 No. 2 1984, pp. 482-486.
- ASAE Engineering Practice, EP433 Loads exerted by free-flowing grains on bins ASAE Standards 1991.
- J. W. Carson, R. T. Jenkyn, and J. C. Sowizal, Reliable and Economical Handling of Bulk Solids at Coal-Fired Power Plants, Bulk Solids Handling 12 No. 1, Feb. 1992, pp 11-18.
- G. Gurfinkel, Tall Steel Tanks: Failure, Design, and Repair, ASCE Journal of Performance of Constructed Facilities, Vol. 2, No. 2, May 1988, pp. 99 to 110.
- H. Purutyan, K. E. Bengtson, and J. W. Carson, Identifying and Controlling Silo Vibration Mechanisms: Part I, Powder and Bulk Engineering 8 No. 11, Nov. 1994, pp 58-65.
- H. Purutyan, K. E. Bengtson, and J. W. Carson, Identifying and Controlling Silo Vibration Mechanisms: Part II, Powder and Bulk Engineering 8 No. 12, Dec. 1994, pp 19-27.
- J. W. Carson and R. T Jenkyn, How to Prevent Silo Failure with Routine Inspections and Proper Repair, Powder and Bulk Engineering 4 No. 1, January 1990.