The capabilities of laser diffraction systems go beyond particle-size analysis to also predict a catalyst’s propensity to experience attrition
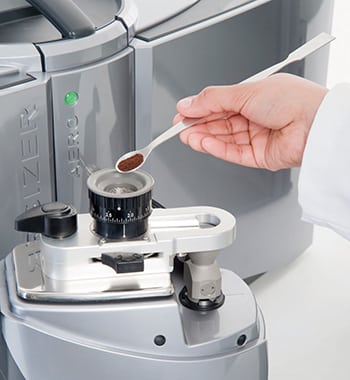
FIGURE 1. By enabling the close control of dispersion conditions during measurement, a laser diffraction system generates accurate particle-size data and also allows an assessment of the propensity of catalyst particles to undergo attrition
Heterogeneous catalysts, where the catalyst is in solid form, enhance many of the gas- and liquid-phase reactions that underpin common operations in the chemical processing industries (CPI). Prime examples include Raney catalysts for the hydrogenation of liquid fats, fluid catalytic cracking (FCC) catalysts for hydrocarbon processing and three-way catalysts for the in-situ treatment of car exhaust fumes. The extent to which such catalysts enhance reaction rates is directly dependent on the specific surface area they present to the reactants, which for particulate catalysts is a function of particle size. Mass for mass, samples with a finer particle-size distribution present a higher surface area and promote faster reactions.
In many instances, tailoring particle size is an important part of the development and manufacture of heterogeneous catalysts. While finer particles may be advantageous from the point of view of reaction potential, downsides include health and safety issues, poor fluidization properties and a tendency to agglomerate, which inhibits the reaction. Optimizing particle-size distribution is therefore essential.
This article examines the role of both wet and dry laser-diffraction particle-size measurement techniques, highlighting the useful information that can be generated within this context. Strong correlations between Brunauer-Emmett-Teller (BET) physisorption data, which are widely used for catalyst characterization, and surface area values derived from particle-size measurement strengthen the applicability of laser diffraction in this area. However, the application of laser diffraction systems (Figure 1) extends beyond size and surface area measurements. Assessments of the tendency of a material to break up or attrite during fluidized-bed processing, for example, are also among the potential applications.
Particle size in catalyst analysis
A catalyst is defined as a material that can be used to alter the rate of a reaction without itself being changed. In heterogeneous catalysis, liquid or gas reactants come into contact with the solid surface of the catalyst, lowering the energy required for a reaction to take place and stimulating increased rates of reaction. Crucial to the success of many industrial processes, the production of effective heterogeneous catalysts relies on closely tailoring a catalyst’s physical properties to meet the requirements of specific reaction chemistry. Within this context, particle-size information is recognized as critical to attaining the desired performance.
The fact that a decrease in particle size results in a directly proportional increase in surface area is of great importance in heterogeneous catalyst properties. It is for this reason that the majority of these catalysts exist in powder form, maximizing the material’s surface area. However, while reducing particle size is an important strategy for increasing catalyst reactivity, not every effect will be beneficial.
Creating a catalyst with too fine a particle size may lead to significant operational issues. Excessive fines are a common cause of poor fluidization behavior, and tend to increase the risk of contamination or pollution issues, in both gaseous and liquid-phase processes. Any resultant catalyst loss also has economic consequences, since catalyst materials often include expensive metals and can carry significant unit cost. In addition, fine particles may be associated with uncontrolled agglomeration, effectively reducing the material’s surface area. Thus, catalyst production is often an exercise in compromise, balancing the need to maximize efficacy with the need to reduce the risk associated with overly fine particle size.
Specific surface area (SSA) is a critical measurement for defining catalyst activity and achieving an optimal particle-size distribution. Traditionally, this has been performed using BET physisorption techniques. However, catalyst specialists are increasingly turning to particle-sizing techniques for fast and efficient assessment. To this end, laser-diffraction particle sizing is finding widespread application for the characterization and development of catalyst powders. Beyond particle-size measurement, this technology has broader applications for optimizing activity through the understanding it provides of the propensity of particles to experience attrition under operational conditions.
Wet and dry dispersion
Laser diffraction is an ensemble particle-sizing technique that delivers a particle-size distribution for the entire sample. Particles illuminated by a laser beam scatter light over a range of angles, with large particles generating high scattering intensity at narrow angles. Smaller particles result in a lower-intensity signal at much wider angles. It is therefore possible to calculate the particle-size distribution of a sample from the scattered light pattern through the application of an appropriate model of light behavior, ideally the Mie theory [ 1 ]. For catalyst applications, the particle-size data that are generated can be used to calculate the SSA distribution for the product.
An important element of laser-diffraction particle-size measurement is to ensure that the sample is dispersed appropriately for the measurement application. This requires taking into consideration the degree to which agglomerates are formed within the sample and whether the measurement data required are for those agglomerates, or, as is more usually the case, for the primary particles in their de-agglomerated state. Applying appropriate dispersion methods ensures that the data generated are truly representative of the powder, within the given application.
Wet dispersion is one technique used to disperse agglomerates within a sample. It involves wetting the powder with a suitable liquid to lower the surface energy between particles. Agitation or ultrasound techniques are then applied to promote agglomerate separation. This technique gently but effectively disperses a sample, and the results provide a baseline for laser diffraction measurements.
The alternative is dry dispersion. This method avoids the use of solvents and wetting agents, making it inherently more environmentally benign and also much faster. Here, dispersion is achieved by entraining particles in a compressed stream, inducing de-agglomeration through particle-particle collision, particle-wall collisions, or by agglomerate shearing during rapid particle acceleration and deceleration.
As with wet dispersion, the target is to disperse the sample to an application-relevant degree, but no further. However, dry dispersion has the potential to cause destructive particle breakup and attrition if excessive pressure is applied to the sample. This potential is recognized in the recommendation to perform a “pressure-particle size” titration, as part of dry-method development, to identify an operating region over which particle size is constant and closely comparable to results from wet measurements [ 2 ].
Interestingly, this capability of the dry-dispersion engine within a laser diffraction analyzer to quantify the level of particle breakup over a range of pressures raises the possibility of using a laser diffraction analyzer to assess the risk of attrition and predict the life of a catalyst under operational conditions in a fluidized-bed reactor, for instance. The development of precise dry powder-dispersion systems that offer sensitive and repeatable dispersion control over a range of pressures delivers enhanced performance in this area, thereby increasing the potential value of laser diffraction systems for catalyst producers.
Measuring specific surface area
The particle-size distributions of three different FCC catalysts were measured using wet-dispersion laser diffraction on a commercially available laser diffraction instrument. The dispersion conditions for these samples were optimized following standard wet-method development strategies [ 3 ].
The results (Figure 2) show how the samples, which all have a median particle size between 60 and 80 μm, differ in terms of their particle-size distributions. While catalyst A and C are relatively similar, catalyst B has a markedly narrower size range. Table 1 shows the SSA of the three FCC catalysts tested, as determined from the laser diffraction data.
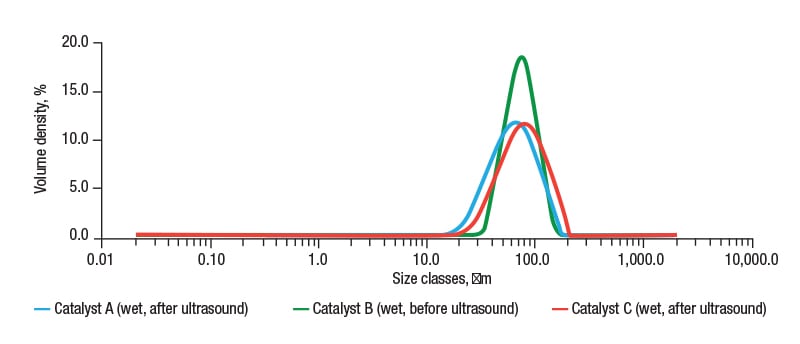
FIGURE 2. Particle-size distributions for FCC catalyst samples A, B and C measured with wet dispersion show sample B to have a much narrower particle-size distribution
These results indicate that the SSA of catalysts A and C are similar, whereas catalyst B has a much smaller SSA. The higher SSA for samples A and C are attributed to the higher levels of fine material present within these products, which are absent from catalyst B.
Figure 3 shows a comparison between the laser diffraction SSA results and analogous data produced using BET techniques. The results show excellent correlation with one another, indicating that laser diffraction can be used in place of BET to predict likely catalytic activity or provide a method for quality-control analysis.
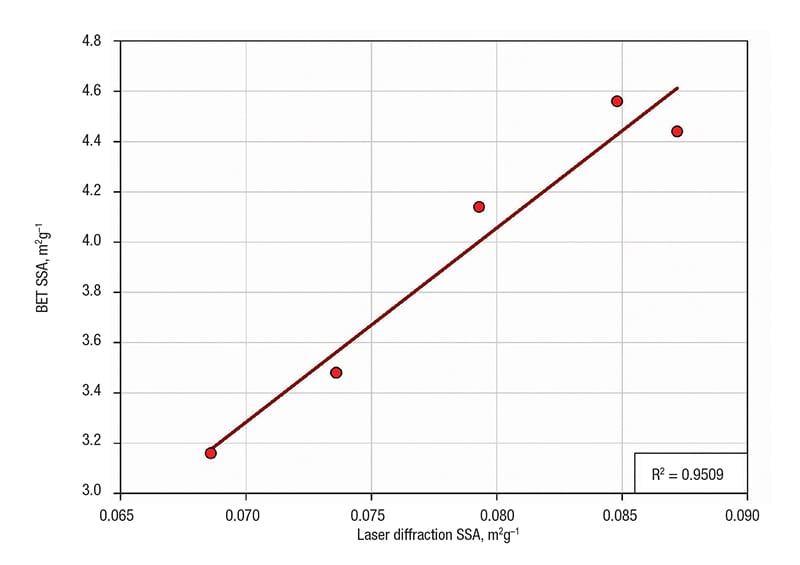
FIGURE 3. A comparison of specific surface area (SSA) derived with Brunauer-Emmett-Teller (BET) data and laser diffraction indicates linearity, confirming laser diffraction as a viable alternative to BET
Predicting attrition
Further investigation of the catalyst samples was carried out to demonstrate the ability of the dry dispersion engine of the laser diffraction instrument to quantify the propensity of the catalysts to undergo particle attrition.
Figure 4 shows the results of a pressure titration for FCC catalyst C. At low pressure, the results show large agglomerates, meaning full dispersion has not been achieved. As the pressure is increased, there is a clear decrease in particle size. At 1 bar pressure, the results show excellent corroboration with the wet dispersion data, indicating complete dispersion has been achieved. Above this pressure, any decrease in particle size can therefore be attributed to particle attrition.
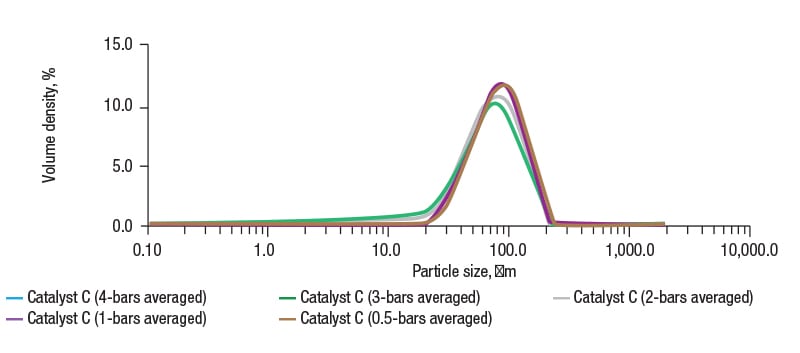
FIGURE 4. Particle-size distribution data for FCC catalyst C measured using dry dispersion laser diffraction at a variety of pressures show that at 1 bar pressure, the particle size corroborates with data derived from wet dispersion for the same powder, indicating complete dispersion
An important parameter in particle-size measurement is Dv10, the size below which 10% of the particle population lies on the basis of volume. The variation of Dv10 with increasing dispersion pressure is most critical, as Dv10 is the most sensitive marker of increases in fine particle fraction caused by attrition. Figure 5 shows Dv10 as a function of pressure for each of the FCC catalysts. These data have been used to calculate an attrition index (AI), which compares the Dv10 measurement at low pressure to that measured at high pressure.
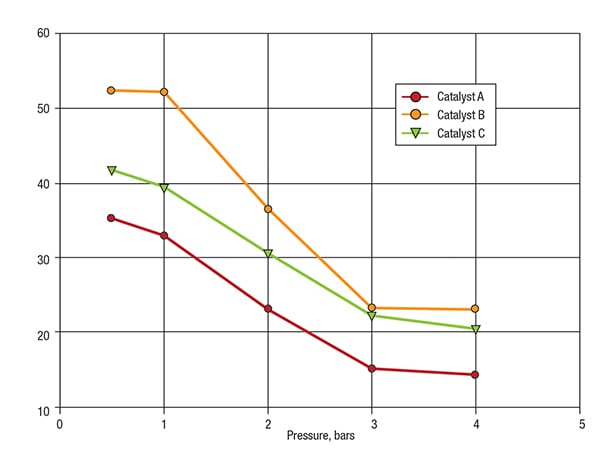
FIGURE 5. Dv10 versus pressure data for catalysts A, B and C show the attrition of particles at increasing pressures
Table 2 shows the AI values calculated for each of the FCC catalysts. These results confirm that A and C exhibit similar attrition behavior, suggesting that they would be likely to have a similar lifetime within a fluidized bed. Catalyst B, on the other hand, displays a more negative AI, suggesting that is more prone to attrition and is likely to have a shorter lifetime than A or C.
Final remarks
These results demonstrate that while calculating specific surface area, laser diffraction analyzers with sufficiently sensitive dry-dispersion engines also offer a valuable opportunity to quantify relative attrition rate, providing useful information for assessing the likely performance of a catalyst within a fluidized bed.
Understanding particle size and specific surface area is crucial to optimizing catalyst activity and efficacy, a necessity that is increasingly being supported by laser diffraction technology. Additionally, the ability to easily monitor the reduction of particle size caused by dry dispersion under different conditions has led to the application of this technique to assess particle attrition to simulate catalyst behavior under operating conditions. In combination, these two capabilities make modern laser diffraction systems a valuable proposition for heterogeneous catalyst characterization as the presented data demonstrate. ■
Edited by Mary Page Bailey
References
1. Mie Theory: The First 100 Years, Malvern Instruments Ltd., 2009, www.malvern.com/en/support/resource-center/whitepapers/wp100108mietheoryhundred.aspx.
2. ISO 13320:2009: Particle size analysis — Laser diffraction methods, Oct. 2009.
3. Wet method development for laser diffraction measurements, Malvern Instruments Ltd., 2011, www.malvern.com/en/support/resource-center/technical-notes/tn130222wetliquiddispersionmethoddevelopment.aspx.
Author
Alan Rawle is applications manager at Malvern Instruments Inc. (117 Flanders Rd., Westborough, MA 01581; Phone: 508-768-6434; Email: [email protected]). In this role, he is responsible for customer support, including training, provision of application notes and other materials, such as peer-reviewed papers. He has more than 30 years of experience in various aspects of particle-sizing technology, and has spent many years working on the ISO TC24/SC4 (Particle Characterization) committee, which has been responsible for standards dealing with laser diffraction and dynamic light scattering. He also led a small international group that developed the international standard for small-angle X-ray scattering (ISO 17867:2015). Rawle is currently co-chair of E 56.02, the characterization subcommittee of the ASTM E 56 Committee on Nanotechnology. In 2014, he was awarded an ASTM Distinguished Service plaque for exemplary leadership. He is also a Fellow of The Royal Society of Chemistry and holds a Ph.D. in supported metal catalysis.