Corrosion-induced failures are frequent in the electronics products used in control rooms, but proper environmental assessment, control and monitoring can help abate these concerns
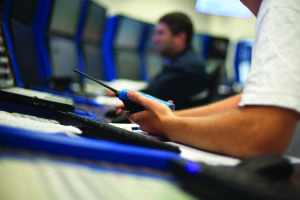
FIGURE 1. The critical electronic components in CPI control rooms are susceptible to corrosion, and protection mechanisms should be put into place to prevent failure.
Electronic process-control equipment — from small remote sensors and instruments to plant-wide distributed control systems (DCS) — make plants in the chemical process industries (CPI) capable of higher production rates, and keep them more competitive in the world market (Figure 1). Chemical, physical and environmental monitoring and control of processes, which previously was performed manually or through simple control systems, is now accomplished in realtime via sensors and actuators linked by a sophisticated DCS. This type of high-speed network enables manufacturers to produce goods economically at high rates and with high quality.
The key to proper control is a properly functioning DCS. This ensures correct sensor function, communications and process control. The corrosive gases that are present in many industrial facilities will permeate into control rooms, DCS spaces and computer facilities, and will most likely degrade and eventually incapacitate critical electronic equipment. This scenario may, in many cases, lead to catastrophic failure in critical control and safety systems. The environment must be properly managed for safe and successful system operation and reliability. This article covers the ways environmental assessment, monitoring and control can be applied to avoid corrosion issues in control rooms.
To begin with, plant operators must manage several environmental species of airborne contamination, including the following:
- Liquids, including small levels of condensation and sea salt mist
- Solids, including grit, sand and dust
- Gases, including active sulfur, sulfur oxides, gaseous chlorine, nitrogen oxides, hydrogen fluoride, ammonia, ozone and strong oxidants
![FIGURE 2. These corrosion guidelines were developed by various collaborating companies to protect control equipment against corrosion [3]](https://www.chemengonline.com/wp-content/uploads/2017/01/36_1.jpg)
FIGURE 2. These corrosion guidelines were developed by various collaborating companies to protect control equipment against corrosion [3]
One complicating factor that has arisen in the last several years has been the passage of the European Union (E.U.) Restriction of Hazardous Substances (RoHS) directive regarding the use of certain hazardous substances in electrical and electronic equipment [ 1]. The RoHS directive restricted the use of lead (Pb), mercury (Hg), cadmium (Cd), hexavalent chromium (Cr 6+), polybrominated biphenyls (PBB) and polybrominated diphenyl ether (PBDE) in the manufacturing of certain electrical and electronic equipment sold in the E.U. Although a misnomer, these regulations are most commonly referred to as “lead-free” manufacturing, due to the fact that essentially all electrical and electronic equipment to this point contained lead. This was the first of many regulations passed to control electronic waste by eliminating lead and other hazardous substances in electronic products. In its first iteration, RoHS provided exemptions for several types of critical electrical devices, including process measurement and control equipment, due to lingering reliability concerns when these regulations took effect in 2006.
The RoHS 2 directive (or RoHS recast) is an evolution of the original directive [ 2]. It became law in the E.U. in July 2011 and took effect in January 2013. RoHS 2 addresses the same substances as the original directive, while the new scope has been extended to all electrical and electronic equipment, including monitoring and control instruments, with full implementation required by July 2017. However, even with this extension, experience has shown that printed circuitboards (PCBs) made using lead-free materials can be more susceptible to corrosion in high sulfur environments, which typically have been associated with industrial applications.
Causes of corrosion
Corrosion of metals is actually a chemical reaction caused primarily by attack of gaseous contaminants, and is accelerated by heat and moisture. Rapid shifts in either temperature or humidity cause small portions of circuits to fall below the dewpoint temperature, thereby facilitating condensation of contaminants. Relative humidity (RH) above 50% accelerates corrosion by forming conductive solutions on a small scale on electronic components. Microscopic pools of condensation then absorb contaminant gases, which become electrolytes in the pools, where crystal growth and electroplating occur. Above 80% RH, electronic corrosive damage will occur, regardless of the levels of contamination.
In the context of electronic equipment, corrosion is defined as the deterioration of a base metal resulting from a reaction with its environment. More specifically, corrosive gases and water vapor coming into contact with a base metal result in the buildup of various chemical reaction products. As the chemical reactions continue, these corrosion products can form insulating layers on circuits, which can lead to thermal failure or short-circuits. Pitting and metal loss can also occur.
Corrosive gases. Specifically, we are only concerned with the three types of gases that are the prime culprits in the corrosion of electronics: acidic gases, such as hydrogen sulfide, chlorine and hydrogen fluoride, as well as oxides of sulfur and nitrogen; caustic gases, such as ammonia; and oxidizing gases, such as ozone. Of the gases that can cause corrosion, the acidic gases are typically the most harmful. For instance, it takes only 10 parts per billion (ppb) of chlorine to inflict the same amount of damage as 25,000 ppb of ammonia. Each site may have different combinations and concentration levels of corrosive gaseous contaminants. Performance degradation can occur rapidly or over many years, depending on the particular concentration levels and combinations present at a site.
The “lead-free” transition. A typical failure mechanism of electronic systems in these environments is the reaction of atmospheric sulfur with exposed metals — particularly copper and silver. These metals are found in printed circuitboard traces, integrated circuit (IC) leads and device terminations. Copper sulfide (Cu 2 S) or silver corrosion products can grow and creep across surfaces like IC packages and printed circuitboard substrates.
However, one failure mechanism caused by the lead-free transition was not foreseen by the industry — products with an immersion silver (ImmAg) surface finish will creep-corrode in what some electronic equipment manufacturers consider to be high-sulfur environments. The number and types of corrosion failures have increased since the implementation of RoHS. The most frequent failures are with the most common components, which include hard disk drives (HDD), graphic cards, motherboards, dual inline memory modules (DIMMs), capacitors and transistors.
Historically, the use of silver in electronic assemblies has been a reliability risk unless the silver is protected from the environment. Silver creep corrosion (electromigration) can occur quite readily in humid environments, especially in the presence of small amounts of atmospheric sulfur compounds and chlorides, which are common in many industrial environments. More than thirty years ago, DCS manufacturers, such as Digital Equipment Corp. (DEC), ABB and Honeywell, described their concerns regarding equipment corrosion in research documents, technical papers and site planning guides. These companies also provided guidance in terms of the environmental conditions necessary to protect their computer equipment (Figure 2) [ 3].
Manufacturers of industrial computer equipment still specify the control of corrosive gases in their site planning guides or in the terms and conditions necessary to maintain warranties and service contracts. Even in environments previously considered benign with regard to electronics corrosion, serious problems as a direct result of RoHS compliance are being reported.
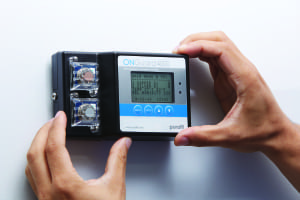
FIGURE 4. Atmospheric corrosion monitors (ACMs) provide realtime data on the performance of the corrosion-prevention measures in a control room, including chemical-filtration and air-quality systems
Contamination control process
With the changes to process measurement and control equipment mandated by the various RoHS directives, process, facility and instrumentation engineers should include environmental contamination monitoring and control as part of overall site planning, as well as risk management, mitigation and improvement plans. This plan should consist of the following three steps:
- Consider the assessment of the outdoor air and indoor environment with regard to corrosion potential. ANSI/ISA Standard 71.04-2013 (hereafter ISA 71.04) can be used to provide site-specific data on the types and levels of gaseous contamination in the amount of corrosion being formed. Corrosion classification coupons (CCCs, Figure 3) can be used as a survey tool to establish baseline data necessary to determine if and what type of environmental controls are needed.
- Develop and specify a targeted contamination-control strategy. Corrosion in an indoor environment is most often caused by a short list of chemical contaminants or a combination of contaminants. The contaminants present in a specific area are highly dependent on the controls put in place to mitigate them. These efforts mainly involve the selection and application of the appropriate chemical filtration systems to clean both the outdoor air being used for pressurization and ventilation, as well as any recirculation air.
- Establish a realtime environmental-monitoring program based on the severity levels established in ISA 71.04. Realtime atmospheric corrosion monitors (ACMs, Figure 4) can provide accurate and timely data on the performance of the chemical filtration systems as well as the room air quality.
The absence of gaseous contamination controls can be the result of a lack of knowledge and education. Often, the relationship between corrosion levels and hardware failures is overlooked or unknown. However, due to the continuing efforts of automation and control companies, this knowledge gap is shrinking, and successful corrosion monitoring and control programs are being developed and implemented, assuring reliable operation of process measurement and control equipment.
Environmental assessments. A simple quantitative method to determine the airborne corrosivity in a control room environment is by “reactivity monitoring” as described in ISA Standard 71.04-2013: Environmental Conditions for Process Measurement and Control Systems: Airborne Contaminants. Copper and silver sensors are exposed to the environment for a period of time and quantitatively analyzed to determine corrosion-film thickness and chemistry. Silver-reactivity monitoring must now be used as part of an assessment in order to provide a complete accounting of the types of corrosive chemical species present in the local environment and to establish environmental severity levels.
ISA 71.04 classifies four levels (Class G1, G2, H3 and GX) of environmental severity for electrical and electronic systems providing a measure of the corrosion potential of an environment (Table 1). The overall classification is based on the higher of the total copper and silver reactivity rates.
Corrosion monitoring. CCCs can be placed throughout the control room to determine compliance with air-quality specifications. Realtime ACMs are used in the controlled environment and on or in server cabinets to provide realtime data on corrosion rates and the effectiveness of corrosion control strategies. Proper assessment will require monitoring of the outdoor (ambient) air and at various locations inside and outside the control center. Either monitoring technique may be used to provide the data necessary to troubleshoot and mitigate contamination issues inside the control center. There are many options that can be considered with respect to air-quality monitoring for industrial control-room applications, and there should be some consideration for room size and layout to determine the minimum number of CCCs and ACMs and their locations, to provide a statistically valid environmental assessment.
There is general confidence in being able to identify contaminant types, for instance active sulfur, sulfur oxides and inorganic chloride compounds, when using corrosion monitoring. This carries more weight from a scientific standpoint in that it can be verified using independent sources of environmental data, such as air-pollution indices or satellite data, to verify the results obtained from corrosion monitoring.
CCCs are typically used for an initial survey of ambient air quality and the environment in which electronic equipment is located, and can be used on a continuing basis to provide historical data. This is especially important where equipment warranties specify establishing and maintaining an ISA Class G1 environment. Realtime monitoring may also be used, but should be limited to the control-room environment. Where corrosion problems have been identified, it is recommended to start with ACMs in a number of locations in order to determine if contamination is widespread or limited to a specific area. Once a baseline had been established, some of the monitors could be later redeployed around the problem areas to gauge the effectiveness of contamination control strategies that may be put in place. Once the environment is under control and meets the conditions set forth in the manufacturers’ warranties, one can determine the best permanent ACM locations for specific needs.
RoHS and reliability
The requirement for corrosion control in industrial environments remains constant. However, more companies are now taking a much closer look at developing or updating specifications due to the changes made by controls manufacturers to comply with RoHS restrictions on the use of lead. This includes specifying an ISA Class G1 environment for control rooms, where in the past, a Class G2 environment was considered acceptable. Specifications are also now emerging that require the measurement and quantification of both copper and silver corrosion rates according to ISA 71.04-2013, with environmental severity levels determined according to the higher of the two.
Although the amount of silver on printed circuitboards and other electrical components has been reduced by many control systems manufacturers, the use of silver is still required and will be for the foreseeable future. With this comes an increased concern over equipment reliability when using a copper-only environmental classification system.
Example: problems with RoHS-compliant equipment. A recycled-paper mill began a project to replace obsolete equipment that could no longer be supported with new DCS and programmable logic controls (PLCs). The original hardware had been installed for 15 years, and had proven to be reliable and robust. Many of the locations where this equipment had been installed were classified as ISA Class G1 for copper. However, the corresponding silver corrosion rates were up to 20 times higher, and essentially all of the silver corrosion reported from the analysis of CCCs collected over several years was due to sulfur corrosion. This presented some concern based on changes to the equipment due to RoHS compliance.
Within three months of replacing the old systems, the mill began experiencing frequent failures of the input/output (I/O) stations installed on the process fieldbus (Profibus). A great deal of work was put into making the fieldbus installations error-free, and the architectures were reengineered to follow the core rules precisely.
Since the problems were intermittent, and symptoms appeared related to Profibus communication or installation, a full diagnostic process was implemented to capture any and all information about each failure. This included examination of the hardware, the hardware location, its position on the fieldbus, architectures and software settings. Through systematic investigation and analysis, several potential causes were eliminated and a root-cause analysis for the remaining issues was performed.
Mill staff recognized that the resistance of a choke installed on the base unit of the I/O station to eliminate signal noise was increasing, and on failed units was a high or open circuit. Ironically enough, this choke was only installed to meet other E.U. legislation pertaining to radio-frequency interference (RFI) emissions. The critical issue identified pertaining to this choke was that it was an electronic chip design with a very isolated thin layer of silver in compliance with the lead-free regulations of RoHS. Corrosion of this silver layer destroyed the choke’s connection to the printed circuit, resulting in the high resistance. Consultation with the manufacturer confirmed the presence of silver on the chokes.
The mill was already in the process of installing air-cleaning equipment to remove corrosive gases from the air and reduce the rates of copper corrosion. However, the extremely short time to failure for these silver-containing components accelerated this effort in order to prevent additional failures. The staff was confident that these failures were purely the results of RoHS compliance and the use of silver. The lack of information and warnings by the supplier regarding potential issues related to their RoHS compliance programs is troubling, considering that even when presented with this evidence, they still concluded these failures were due to “installation errors.”
Problems exist in many locations. CCC data from several additional mills (Table 2) show that many locations exhibiting an ISA Class G1 environment for copper, including air-handling units (AHUs) and motor control centers (MCC), have corresponding silver corrosion rates that would now cause the environment to be classified as G2, G3 or even GX. This would indicate serious cause for concern for any electronic equipment with an ImmAg surface finish specifically or any silver or silver-plated components in general.
Another contributing factor to concerns over the increased use of silver in industrial applications is that even with tightened control requirements for other environmental parameters (including temperature and humidity) and the positive effect this has on the rate of copper corrosion, silver can still exhibit high rates of corrosion, even in well-controlled environments. Closer examination of CCC data shows that in locations reported as ISA Class G1 for copper corrosion, the corresponding silver corrosion rate can be up to 10 times higher [ 4]. Furthermore, every CCC analyzed shows evidence of sulfur contamination (as Ag 2 S). On average, the amount of silver corrosion measured is double that of the copper corrosion reported.
Contaminant gases containing sulfur, such as SO 2 and H 2 S, are the most common gases that cause hardware corrosion in paper mills, petroleum refineries and chemical plants, and corrosion control is acknowledged as a requirement to assure electrical and electronic equipment reliability. One example of component failure is from sulfur gases entering a component package and attacking the silver, resulting in the formation of Ag 2 S. The mechanical pressure created by the Ag 2 S formation inside the package damaged its mechanical integrity and caused the device to fail. This and other failure mechanisms are becoming common occurrences when using RoHS-compliant electronic equipment and components produced using the ImmAg process, and to a lesser degree, the electroless nickel-immersion gold (ENIG) process.
To maintain a high level of equipment dependability and availability, it should be understood that a control room is a dynamic environment where many maintenance operations, infrastructure upgrades and equipment change activities occur on a regular basis. Airborne contaminants that are harmful to sensitive electronic devices can be introduced into the operating environment in many ways in addition to the ventilation system. For instance, chlorine can be emitted from PVC insulation on wires and cables if temperatures inside the DCS cabinets get too high. However, it is still the outdoor ambient air used for cooling and pressurization that remains the primary source of corrosive contaminants, and this air should be cleaned before its introduction into the control-room environment.
With the changes to process control equipment due to the RoHS directives, plant managers and operators should include an environmental contamination monitoring and control section as part of an overall site plan, as well as plans for risk management, mitigation and site improvements.
Looking forward
RoHS regulations, along with the continuing reductions in circuitboard feature sizes and miniaturization of components necessary to improve hardware performance, makes today’s electronic hardware more prone to attack by airborne contaminants. Increases in corrosion-related electronic hardware failures have led to new electronic equipment warranties that require environmental corrosion (reactivity) monitoring and control of airborne contamination where necessary.
Manufacturers have to maintain the reliability of their equipment, and therefore the need to control airborne contaminants and to specify their acceptable limits are now considered to be critical to the continued reliable operation of process-control equipment. ANSI/ISA Standard 71.04-2013 now includes silver corrosion monitoring as a requirement in determining environmental severity levels. Most manufacturers of process-control equipment currently reference this standard in their site planning and preparation guidelines, as well as their terms and conditions for warranty compliance. The addition of silver corrosion rates as a required metric serves to bridge the gap between ambient environmental conditions and the reliability of RoHS-compliant (lead-free) electronic equipment.
Ongoing research will serve to further refine Standard 71.04 both quantitatively and qualitatively. This, along with continuing advancements in the monitoring and control of corrosive contaminants, will help to prevent costly and potentially catastrophic failure of critical electronic equipment.
The requirement for the control of corrosive gases in the CPI remains constant. Assuring longterm equipment reliability requires working with plant personnel to quantify the corrosive potential of an environment toward the various types of electronic equipment in use, providing engineered solutions for gaseous contaminant control and ongoing monitoring of the controlled environment to assure compliance with standards and specifications. The successful application of such a corrosion control program in CPI settings will protect critical process measurement and control equipment and assure profitable manufacturing operations. ■
Edited by Mary Page Bailey
References
1. European Union (E.U.) Directive 2002/96/EC “Of the European Parliament and of the Council of 27 January 2003 on waste electrical and electronic equipment (WEEE),” Official Journal L 37, Feb. 13, 2003, p. 24.
2. European Union (E.U.) Directive 2011/65/EU “Of the European Parliament and of the Council of 8 June 2011 on the restriction of the use of certain hazardous substances in electrical and electronic equipment (recast),” Official Journal L 174, July 1, 2011, p. 88.
3. Digital Equipment Corp., Copper Corrosion Rate Distribution Guidelines, 1985.
4. Purafil Corrosion Classification Coupon (CCC) Database, 2015.
Author
Chris Muller is the technical director for Purafil, Inc. (2654 Weaver Way, Doraville, GA 30340; Email: [email protected]), a manufacturer of gas-phase air-filtration media, filters, equipment and air monitoring instrumentation. He is an expert on environmental air quality and gas-phase air filtration, and has helped develop many standards on the topic of air filtration. He is an ASHRAE (American Society of Heating, Refrigerating and Air-Conditioning Engineers) Distinguished Lecturer who has presented over 75 seminars. Muller has written over 150 peer-reviewed papers and articles.