Manufacturing complexes may benefit from using more electricity instead of steam and fuel gas for their energy needs. Improved energy efficiency, lower emissions, higher reliability and debottlenecking of utility systems may be achieved
Electrification is a popular buzzword when energy transition is discussed. It can have many different meanings, and depending on the subject, it can refer to the private and business transportation fleet (cars and trucks), public transportation (buses and trains) or manufacturing and industrial processes and facilities.
In process facilities, replacing steam and fuel gas with electricity can provide many benefits, from improvements in reliability to avoidance of boiler-upgrade projects to lower overall emissions. In short, if the carbon footprint from the electricity production is lower than the carbon footprint from producing the energy that is replaced by electricity, then there will be a decarbonization benefit from the electrification.
This article discusses how electrification can impact a process-focused industrial complex, such as a chemical plant or petroleum refinery, and it specifically focuses on applying electrification to existing industrial facilities. For a deeper discussion of the decarbonization potential of electrification projects, please see Part 2o f this Feature Report, Incremental Electrification Drives Sustainability.
WHERE TO BEGIN
There are several questions or issues owners or managers of existing industrial facilities may have when considering electrification of their facilities (Figure 1).
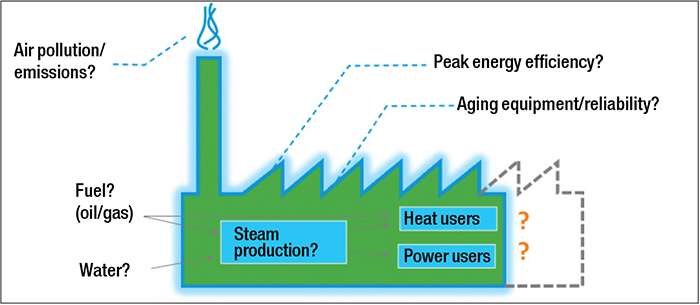
FIGURE 1. There are many questions or issues that owners or managers of existing industrial facilities may have when considering electrification of their facilities
Is the facility operating at its peak energy efficiency? Many operators see a noticeable improvement in their energy intensity index (EII) from electrification of existing steam-turbine drivers. The calculations are based on efficient grid-electricity production, such as from renewable sources, compared to less-efficient boiler steam production.
Improvements in energy intensity are often a part of a facility’s strategic plans and goals, which can have a noticeable impact on its operating costs.
Are the steam-generating systems and turbines in the facility becoming obsolete, and now require ever-growing maintenance needs? Owners may have planned steam-boiler replacement projects or other equipment maintenance or upgrades. If so, owners may want to consider if this investment may be better spent on electrification. Overall equipment reliability may increase and there may be environmental credits or rebates that can be availed with electrification projects.
Is the facility in compliance with local regulations on pollutant emissions? Owners may be facing looming environmental regulations that will require significant investments, such as installation of selective catalytic reduction (SCR) systems for steam boilers or process heaters, to avoid paying hefty fines. An electrification project can reduce steam demand and potentially allow the shutdown of older steam boilers, therefore reducing the cost of any required environmental upgrades. The emissions reductions from the decrease in steam demand resulting from electrification can also be used to offset an increase in emissions from other process heaters with process changes that can be valuable for other strategic goals.
Are the utility systems at capacity, or worse, out of balance? If steam, cooling water or natural gas supply are limited in the facility, it may not be possible to expand or carry out other projects without doing some electrification projects. If the cost to purchase or produce electricity is lower than importing natural gas, then electrification can reduce operating costs.
Is there a need to re-balance the steam and electricity usage, but there are concerns about what to do with excess fuel gas? There are potential synergies in executing an electrification project coupled with a project to increase liquefied petroleum gas (LPG) recovery from the fuel-gas system. Burning less fuel translates into less CO2 generation, which is a strategic driver for many companies. By recovering more LPG and heavier material from the fuel-gas system and replacing it with natural gas, CO2 emissions will be further reduced, even if the fired duty does not decrease. In most markets, the revenue from the sale of LPG products and other liquid products is more than the cost of additional import of natural gas, so there can be financial benefits in addition to CO2 reduction.
Does a facility-wide energy optimization sound intriguing, but also intimidating?If the owner is planning a strategic reconfiguration based on market-driven feed or product changes, that may be an opportunity to replace aging steam-driven compressors with new motor-driven compressors that have a fit-for-purpose duty specification.
ELECTRIFICATION OPTIONS
Once these questions have been considered, operators can begin to weigh their options. For industrial processing facilities, there are three primary electrification options, described below:
- Heat pumps replacing reboilers or condensers for distillation columns
- Electric heating or electric boilers replacing steam or fuel-fired heaters
- Electric motors replacing steam-turbine drivers
These options can have significant impacts in the right situation. Table 1 summarizes the potential impact of the different options to a facility. They are discussed individually in the following sections.
Heat pumps
Heat pumps use a compressor to compress a lower-temperature vapor stream to a high-enough pressure that the condensing temperature is above the temperature required to recover the heat in a traditional heat exchanger. Historically, this design has been called a heat pump, but has also been called mechanical vapor recompression (MVR). This scheme can be used for distillation columns where the column overhead is compressed to a high enough pressure to provide the heat to reboil the bottom of the column. It is also possible to design a distillation heat pump with a separate fluid in a closed loop that is compressed, condensed in the reboiler, further cooled by dropping the pressure across a valve, and then used to cool the overhead condenser. Most examples in the industry use the overhead vapor as the heat-pump fluid, as shown in Figure 2.
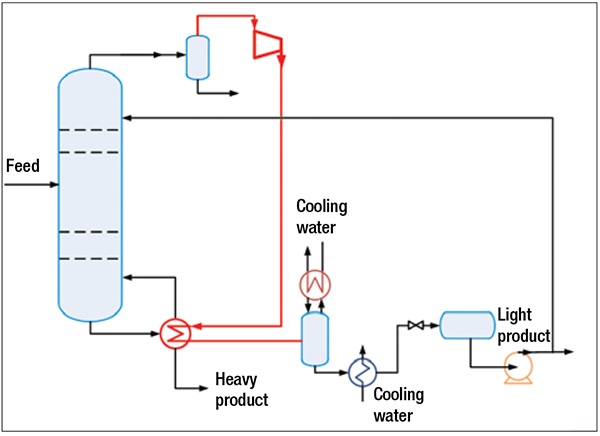
FIGURE 2. A typical heat-pump system on a distillation tower is shown
Heat pumps can have significantly better energy efficiency than traditional reboiler or condenser systems, with savings on both the reboiler side (which often contains steam) and the condenser side (which often contains cooling water). Energy-intensity reductions of up to 90% are reported in the literature.
Heat pumps work very well for columns that do not have a large temperature difference between the top and bottom of the column. Propane/propylene splitters, ethane/ethylene splitters (such as in steam-cracker units), and isobutane/ n-butane splitters are good candidates for heat pumps. There are also applications in aromatics complexes and methanol production.
For most other distillation separations used in refineries and petrochemical complexes, the much larger temperature change across the column significantly reduces the value of the heat-pump approach and makes it impractical.
Heat pumps are best suited for a new design, as it would require a major revamp to retrofit an existing column with a heat pump. This option can reduce consumption of fuel, steam and water, reduce emissions and improve efficiency at the cost of a higher electricity demand.
Electric heaters
There are two ways to electrify steam or fuel-fired heaters, as follows:
- Replace a steam heater with an electrically heated exchanger
- Replace a fuel-fired steam boiler with an electric boiler
To convert a steam-heated exchanger to an electric heater, the steam and condensate piping and controls are replaced with electrical supply, which may reduce maintenance costs and capital costs for a new facility. An advantage of a steam exchanger is that the maximum temperature is self-limiting, meaning that the process fluid cannot be heated to a temperature higher than the condensing temperature of the steam. An electric heater will not have the same intrinsic feature, and controls must be designed to provide protection against overheating the process fluid for upset, low-flow and blocked-in scenarios.
Like the heat pump, this option can reduce fuel, steam and water consumption, reduce emissions and improve efficiency, while increasing power consumption and potentially reducing process-temperature controllability.
Another way to electrify heaters is to replace a fuel-fired steam boiler with an electric boiler. This may be a good option for a revamp of an existing facility because this option reduces emissions from steam generation while still supporting existing process-steam demand, including steam-injection and steam-heating needs.
Electric motors
Several facilities have had positive results from replacing steam-turbine drivers with electric motors. Improved energy efficiency, reduced emissions, improved reliability and lower water consumption have been observed, and some sites have avoided additional steam-boiler investment. The project can impact the power infrastructure, steam, fuel-gas and cooling water systems, as well as potentially impacting process controls and relief systems.
If installing a new pump or compressor, consider if an electric motor is a viable option, even if the previous or typical driver for the service was a steam turbine.
If a facility decides to replace an existing steam turbine on a compressor or pump with an electric motor and there is adequate plot space available, installation of a new pump or compressor along with the replacement motor may not cost more when factoring in the cost savings of doing construction outside of the turnaround. Installing a new pump or compressor also replaces an older pump or compressor that can be optimized for current operations and may result in reduced maintenance and operating costs.
Steam-turbine drivers are often used to drive large compressors. Steam-turbine drivers have an inherent ability to change speeds, which can provide more flexibility and operability for the compressor to manage changes in gas molecular-weight and flow requirements. When considering switching an existing steam turbine driver to an electric motor, a decision will need to be made whether a variable-speed driver is required or if a fixed-speed driver can be used. If a fixed-speed driver is used, the impact on the compressor operation will need to be considered. If the gas molecular weight varies significantly, the compressor capacity and discharge pressure can vary at a fixed speed, potentially causing process and equipment design pressure issues. Relief scenarios and flare loads may also be impacted. Figure 3 and Table 2 illustrate some of the process impacts of motor speed.
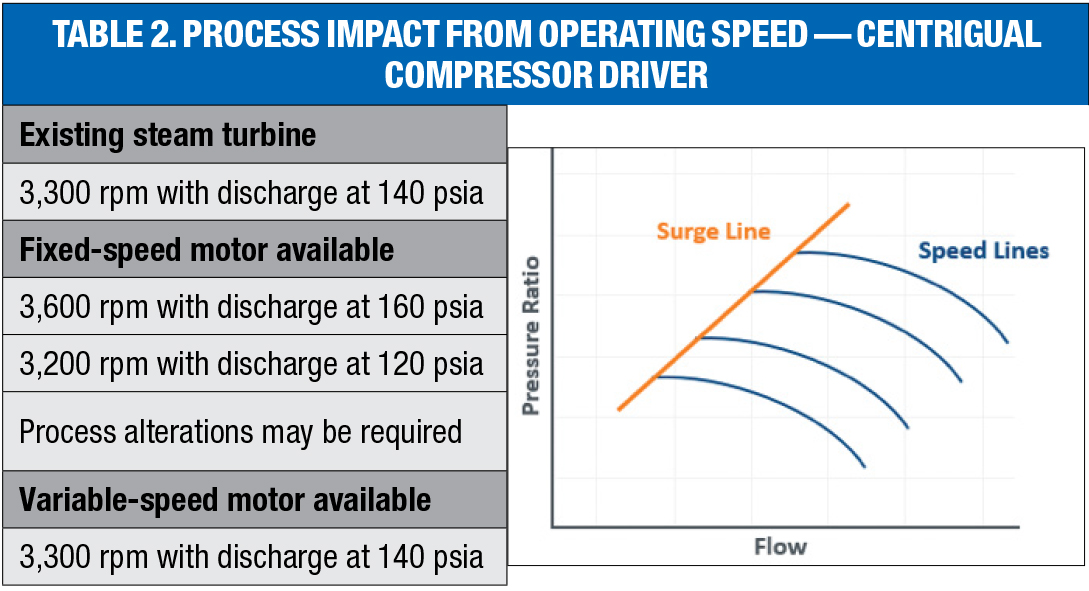
FIGURE 3. Electrification can have a significant impact on processes when considering the available motor operating speeds
Energy consumption may increase in some cases due to excess flow or pressure now produced by the motor-driven compressor. The suction throttle valve and spillback valve may need to be modified or replaced to provide the flexibility that was previously provided by varying the turbine speed.
If a variable-speed driver is required, consider installing a variable-speed motor or installing a fixed speed motor with a variable speed gear box. Installing a variable-speed motor may be an option, but may have a higher cost and larger space requirement for large motors. A better option may be to install a variable-speed gearbox to convert a fixed-speed motor to different speeds as required to optimize the compressor operation.
A knowledgeable engineering team should be consulted to study the impacts of electrification and propose mitigations.
Example: Replacing a steam turbine with a fixed-speed motor.There are two main types of steam turbines — condensing and backpressure. There are also condensing turbines that extract steam at one or more intermediate pressures.
Condensing turbines exhaust steam at a very low pressure — well below atmospheric pressure. The exhaust steam is then condensed, usually in cooling-water exchangers, although air coolers are sometimes used.
Backpressure turbines take a relatively high-pressure steam, as an example, 600 psig, and exhaust it at a lower pressure, for instance, 150 psig. Since backpressure turbines extract less energy from the steam compared to a condensing turbine, more steam is required for a backpressure turbine compared to a condensing turbine producing the same driver load. Table 3 shows an example of how steam demand can be reduced with electrification of an existing steam turbine.
ELECTRIFICATION SYSTEM IMPACTS
For any project, capital cost is an important factor — and often the deciding factor on the feasibility of a project. But there are other factors that should be considered to fully and properly evaluate the impact of an electrification project to the facility.
For an electrification project where steam-supplied energy is being replaced by electricity, the obvious impacts are to the steam system and power supply. Any electrification project is also likely to have secondary impacts to other utilities and systems.
Electrical infrastructure
Electrification will directly impact the electrical system. The existing electrical infrastructure may indeed have the necessary spare capacity to supply the additional power load. However, the reality is that most facilities have limited or no spare capacity, thus requiring some new infrastructure, such as new substations or upgrades to the existing infrastructure.
Electrification loads can range from 5,000 to 20,000 hp, and can be even larger in some cases. Motors of this size have a significant impact on the electrical system, which needs to be studied carefully, as most facilities were not originally designed to support these large additional loads. The following are three key electrical-system studies to be performed to assess the existing electrical infrastructure:
- Load flow — to check capacity of existing equipment to support the new loads
- Short circuit — to check if existing equipment is rated to support additional motor short circuit contribution
- Motor starting — to check if the new motor can be started without impacting the system voltage
These are complex studies that can be performed by an experienced electrical engineer using commercially available electrical-analysis and simulation software, such as ETAP, SKM and Power Factory.
One consideration in starting large motor loads is to apply reduced-voltage starting methods using an autotransformer, capacitor, reactor or even an adjustable-speed drive. Each of these motor-starting schemes have different capital costs, so it is important to evaluate the best option that fits the mechanical or process needs of the load.
Upgrades to the existing electrical infrastructure may include additional utility power, including new transmission lines to take delivery of power at a higher voltage or expanding the site’s cogeneration plant for more power generation (if the facility has one).
The new electrical configuration will impact equipment in both the main and unit substations. Most likely the transformers, switchgear and other power equipment will require upgrades. The new electrical system will likely have dedicated substations for these large loads.
There may also be an impact to the facility’s underground and aboveground electrical infrastructure, as well as remove the associated effects on pipeways and other structural needs to support the new conduit or trays that will feed the new loads.
Lastly, the design must consider construction and cutover challenges. If electrification is done in an existing operating facility, the electrical upgrades need to be well planned. Electrical work requires equipment to be de-energized, so the tendency is to schedule that work during a turnaround (TAR) or during scheduled maintenance to minimize the impact to the operating facility, which may impose a significant constraint on the project schedule.
Steam systems
The electric motors will reduce steam demand when replacing steam drivers. However, it is important to understand the overall impact to the steam balance at the various steam levels. If the facility is long on low-pressure steam, electrification of a backpressure steam turbine will reduce production of excess low-pressure steam. Conversely, if the facility is short on low-pressure steam, electrification of a backpressure steam turbine may not have much value, since the steam saved from electrification will just shift to the high-pressure to low-pressure steam letdown station to balance the low-pressure steam demand.
Electrification of a condensing turbine reduces high-pressure steam demand, which is usually generated in steam boilers. For most facilities, this can be a significant benefit.
Water systems
Reduction in steam demand leads to a reduction in the boiler-feedwater demand, including reduction in treated-water makeup. Water consumption is often a major expense for an operating facility, so this reduction may have a large impact.
The cooling-water system can also be impacted by an electrification project. Many large steam turbines are condensing turbines, which usually have large cooling-water surface condensers. Replacing a condensing steam turbine with an electric motor can lead to a significant reduction in cooling-water demand, which debottlenecks the cooling-water system, potentially supporting other projects that would increase cooling-water demand, and reduces cooling-water makeup consumption. In some cases, an entire cooling tower, or cooling-water pumps, may be shut down, reducing power consumption as well.
Electrification of a condensing steam turbine, however, eliminates production of vacuum condensate, which is often used by some processes. In such cases, an alternate source of clean water will need to be made available for these users.
Fuel-gas systems
The fuel-gas system will frequently be impacted during electrification activities. Fuel-gas consumption is reduced if fired heat is directly replaced by electricity. Likewise, if steam is replaced by electricity, fuel-gas consumption at the steam boilers is reduced. This is beneficial if the fuel-gas system is supplemented by purchased natural gas, because electrification will reduce natural-gas usage, but could be an issue if the facility is long on fuel gas.
Many facilities run close to fuel balance and will be long on fuel gas with major electrification changes. If this is an issue, there may be options for recovering more LPG range and heavier material from the fuel-gas pool, or potentially modifying process-unit operations to produce less fuel gas.
Projects to increase C3+ recovery in gas plants can have a very good payout in selling more LPG product, in addition to the benefits of electrification. If this option is pursued, product storage and transfer logistics will need to be understood so all potential project requirements are addressed.
It may be possible to reduce the amount of heavy material that ends up in the fuel-gas system by making changes in conversion units, such as hydrocrackers and fluid catalytic cracking (FCC) units. Catalyst or operating condition changes can be considered for production of less light-end content from cracking, which ultimately end up in the fuel-gas system.
The potential impact to the fuel-gas heating value should be evaluated with the reduction of LPG material in the fuel gas. Replacing LPG with natural gas high in methane will lower the fuel-gas heating value, and there may be heaters that will experience fuel-gas hydraulic issues with a lower average heating value.
Flare systems
Another impact to consider is to the flare system. The flare load may possibly increase during a local or total power-failure scenario if a turbine-driven pump or compressor not considered to fail previously is replaced by a motor. Conversely, if a steam heat exchanger is replaced by an electric heater, a power failure would cut the heat source that could potentially reduce the total relief load. Total power failure case is often the controlling scenario for the design of the flare and relief header system. Thus, this may be an important factor to consider in the case for electrification.
Electrification considerations
So how can an operator determine what to do?
The first step is to develop an accurate power, steam and fuel-gas balance for the facility. Consider upset and other operating cases, such as when individual units are shut down for maintenance.
Operators should also understand the reliability of the existing systems and what outages will have a large impact on operations.
It is also important to evaluate the planned project portfolio for the facility, to look for cases where projects can facilitate, or be improved by, including electrification as part of the project. As an example, if there are steam-boiler replacement or reliability improvement projects, or pump or compressor replacement projects, there may be synergies for considering electrification as part of those projects.
Understanding the costs for incremental power purchase and import natural gas is helpful. Operators should become knowledgeable about the upgrades the existing systems within the facility will require with an electrification project — but they should also be aware of possible infrastructure impacts to the “across-the-fence” electrical supplier, such as the utility company.
Another crucial factor that must be considered is which current or future emissions requirements (including carbon footprint) impact the facility and would need to be resolved. These could be regulatory-driven or internal company requirements. For example, if the existing steam boilers will require the addition of NOx-removal equipment, electrification may provide an opportunity to build new, smaller, more efficient and more reliable steam boilers for not much more than the regulatory revamp cost. Similarly, if there are steam-boiler reliability upgrade projects planned, decreasing steam consumption instead of implementing a boiler replacement or reliability upgrade project may be a better option.
To find the best project for the facility, start with high-level goals, develop a good understanding of the existing systems, and compare options while understanding which parts are independent that can be evaluated individually and which parts are interrelated and need to be considered as a group.
Clearly, both benefits and costs must be understood.
The potential benefits include:
• Reduction in operating cost
• Improvement in energy efficiency
• Reduction in emissions
Associated costs include:
• Capital cost to implement changes
• System upgrades required (both within the facility and by third-party suppliers)
• Process inside battery limit (ISBL) and outside battery limit (OSBL) impacts, both positive and negative, for each option
Finally, note that it does not help if a preliminary study makes an option look promising, but impacts that were not initially considered make the option much more expensive or impractical. ■
Authors
James Turner ([email protected]) is an executive process director in Fluor Corp.’s Sugar Land, Tex. office. He has more than 30 years of experience in process design for a wide range of projects in the petroleum refining, chemicals and gas-processing industries. He has published and presented many technical articles about process design, project execution, energy transition and decarbonization, and holds patents for a combined hydrotreater process design. He is a graduate of Texas A&M University with a B.S.Ch.E. He currently serves on the Texas A&M Chemical Engineering Department Industry Advisory Council, and has been inducted into the Texas A&M Chemical Engineering Academy of Distinguished Alumni.
Ma Katrina D. Sanaie ([email protected]) is a principal process/specialty engineer in Fluor’s Aliso Viejo, Calif. office. She has more than 20 years of experience in the process design of utilities and offsites for a wide range of projects in the petroleum refining and gas processing industries, and a subject matter expert in flare systems and utilities and offsites. She holds a B.S.Ch.E. from the University of the Philippines and is a registered professional engineer in California.
Mohamad Fakhreddine ([email protected]) is a process engineer located in Fluor’s Long Beach, Calif. office. He has more than 15 years of experience in process design for a wide range of projects in the petroleum refining, utilities and offsites and power-generation industries. He is a graduate of California State Polytechnic University – Pomona with a B.S.Ch.E.