Membrane-supported processes can bring many benefits in water-treatment applications where membranes have not traditionally been used
Reverse osmosis (RO) is the standard treatment technology for purifying water supplies for potable water applications and many industrial processes. One of four crossflow, pressure-driven membrane-separation processes, RO rejects salts, as well as low-molecular-weight dissolved organic compounds. As pressurized water flows over the membrane surface, a portion is forced through the membrane and produces purified water (permeate). This process can be described as filtration on an atomic scale.
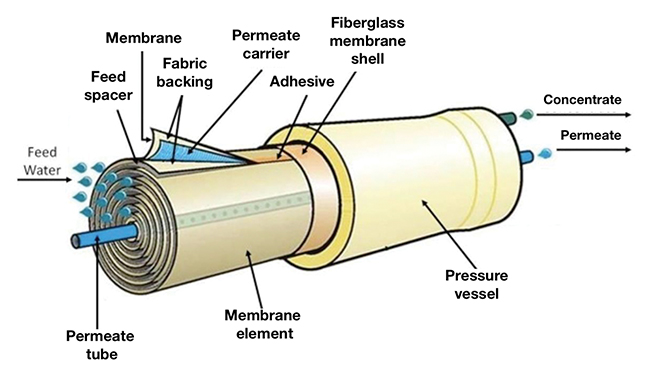
FIGURE 1. The spiral-wound configuration is the most common membrane module used in reverse-osmosis installations
Because of their lower cost, most RO membrane modules (elements) in use today are of the spiral-wound configuration (Figure 1). However, the close spacing of the membrane layers are susceptible to fouling by many water-borne contaminants. This, coupled with the fact that the typical spiral-wound RO membrane element cannot be backwashed, makes them susceptible to fouling of the membrane surface, which is the primary cause of failure in RO processes.
Typically defined by their pore sizes, there are three additional technologies besides RO in the suite of membrane separation processes: nanofiltration (NF), ultrafiltration (UF) and microfiltration (MF). The use and applications of these membranes are a function of the chemical characteristics of the contaminants to be removed and the pressure requirements of each technology. This article outlines the use of newer UF and MF membrane technologies for traditional wastewater-treatment applications.
Water impurities
Although their concentrations can vary widely, the following inorganic chemicals are found in virtually all raw water supplies:
- Calcium
- Magnesium
- Sodium
- Silica
- Chloride
- Bicarbonate
- Sulfate
In addition to the above chemicals, water supplies contain various concentrations of suspended solids, such as soil, dust, clay and others, and often organic contaminants.
Groundwater sources usually have lower concentrations of suspended solids, but higher concentrations of inorganic chemicals, including relatively insoluble compounds containing iron and manganese. Various water sources may concentrate these inorganic elements due to evaporation or from reject streams from other processes. Examples include cooling towers and RO-reject (concentrate) streams.
Because of their relatively low solubilities, calcium, magnesium and silica present problems in many water-treatment applications. Virtually all natural water sources are subject to scaling from calcium and magnesium compounds (often referred to as hardness), and certain locations are subject to silica scaling.
The traditional process to remove chemical compounds that cause hardness is lime softening (also known as lime-soda treatment), which involves the addition of lime (calcium oxide or calcium hydroxide) and soda ash (sodium carbonate) to raise the pH to about 10.5, causing the hardness to precipitate as calcium carbonate and magnesium hydroxide. Because of the reaction time required for complete precipitation to occur (1.5–3 h), large reaction tanks are required. In addition, this process produces a high volume of precipitated calcium carbonate and magnesium hydroxide in a dilute solution (less than 20% solids) usually requiring dewatering and disposal.
The stoichiometric concentrations of the added chemicals depend on the feedwater analysis. However, the treated water will still contain some hardness, usually in the range of 50 to 85 mg/L.
Silica can be effectively removed in this process, usually by coprecipitation with magnesium. Depending on the feedwater analysis, this may require the addition of magnesium chloride and ferric chloride. In many applications, sodium hydroxide is used instead of lime. This is a more expensive chemical — however, it generates less sludge. In industrial wastewater treatment, heavy metals are traditionally treated by raising the pH to create insoluble oxide (or hydroxide) compounds, which are also removed by virtually the same clarification process as described above.
New membrane technologies
An advanced membrane-based process is now being utilized to replace the traditional clarification and settling processes used to remove suspended solids in water-treatment processes. Normally, clarification and settling processes allow the precipitated solids to settle out by gravity. Figure 2 shows a typical gravity settling clarifier.
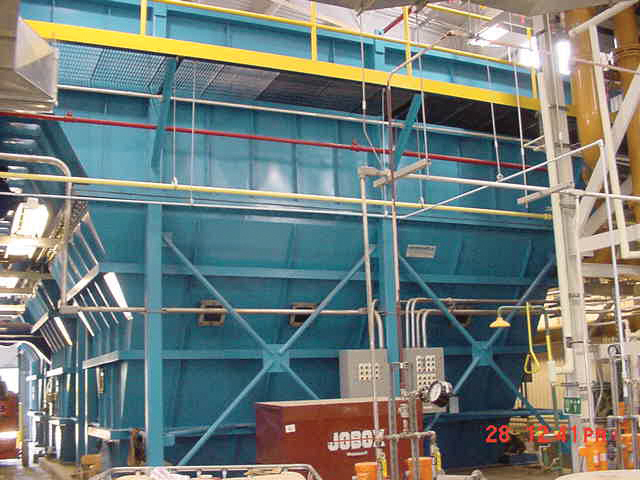
FIGURE 2. A gravity settling clarifier is commonly used to remove suspended solids in many wastewater treatment plants
Conversely, in the new membrane process (Figure 3), the chemically treated solution is recirculated through a tubular MF or UF membrane designed to continuously dewater the stream, producing a filtered water stream (permeate).
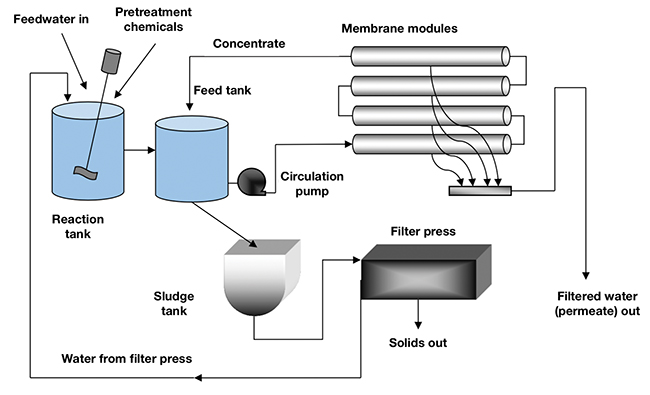
FIGURE 3. A crossflow-membrane system using MF or UF modules can achieve good separation of suspended solids from wastewater
The concentrate stream of the membrane is recycled back to the feed tank to increase the solids concentration to approximately 6%, at which point the stream is discharged into a sludge tank (settler), and from there into a filter press for final dewatering to a solids concentration as high as 80%. The membrane usually has a nominal pore size of 0.10µm and the tubular elements are typically 8-mm diameter and constructed of polyvinylidene difluoride (PVDF) polymer. Figure 4 shows an end view of typical tubular membrane modules.
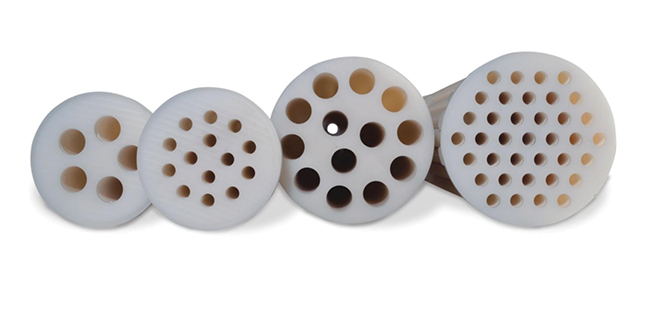
FIGURE 4. Tubular membrane elements can be used in place of a gravtiy clarifier for some water treatment applications
The mechanism of contaminant rejection is size exclusion (absolute filtration) — meaning that what is too big will not go through the membrane. Typically, the filtered material will build up a “gel” layer on the membrane surface, which may actually help to filter out smaller contaminants. The flow of the feedstream across the membrane surface (crossflow velocity) is intended to minimize this layer thickness.
Parameters and performance
Before we can fully compare the performance of traditional clarification and settling systems with membrane-based processes, we must understand the operation of tubular membranes. Figure 5 illustrates the typical operational configuration of a tubular membrane module, and the following are four key parameters that control the performance of tubular membrane-filtration systems:
- Membrane pore-size rating — too large a pore size can lead to rapid mechanical blinding of the pores, resulting in fouling
- Crossflow velocity — if this is too low, an increasingly dense cake (gel layer) can build up on the membrane surface, causing fouling and low permeate flow
- Transmembrane pressure (pump pressure across the membrane) — generally, higher values here produce more permeate flow (flux), but more rapid fouling
- Temperature — increased temperature produces increased flux rate (corresponding to decreased surface tension and viscosity)
Table 1 provides a review of the relationship between various MF membrane parameters. The term “retentate” in Figure 5 identifies the stream that has not passed through the membrane. It contains all of the contaminants rejected by the membrane. This term is synonymous with “concentrate” and “reject.” Figure 6 represents a typical tubular membrane module in cutaway view.
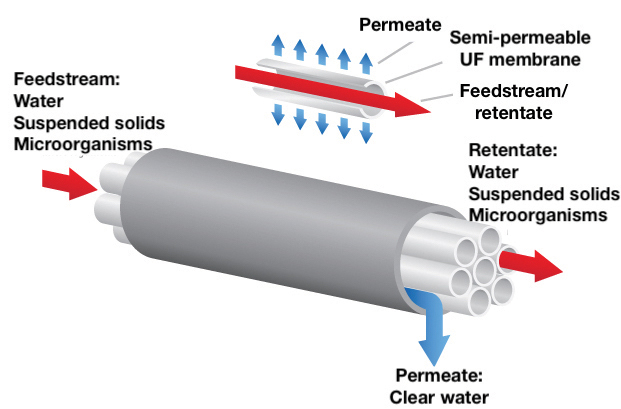
FIGURE 5. There are several operating parameters that impact the performance of tubular membrane-filtration systems
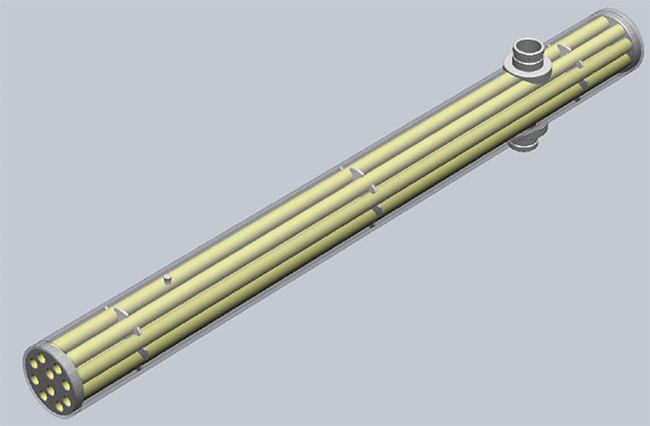
FIGURE 6. The internal elements of a typical tubular membrane module are shown here
Filtration vs. clarification
Because the removal of the precipitated solids in the membrane separation process is absolute filtration (a function of pore size), it is capable of much finer filtration than clarification.
Upsets in clarifiers are well documented and can create events that foul downstream RO processes, often resulting in the membranes requiring chemical cleaning or even replacement. Such issues are nonexistent with the membrane process. The only way that suspended solids can get through the membrane is a catastrophic failure of the MF/UF membrane. This is very unusual.
Below are some additional comparison points between traditional clarification and MF/UF processes:
- Clarifiers require a large footprint. In general, the membrane process footprint is one-third to one-half that of a gravity clarifier
- In most cases, a highly automated MF/UF system is easier to operate and more user friendly than clarification, which requires an experienced operator to maximize the system performance and minimize solids upsets
- A polymer is often used in a gravity settling process to promote flocculation and the formation of a sludge blanket. This is not needed in the membrane process, as solids separation is performed entirely by the membrane
- Capital costs of larger systems are generally higher with the membrane system, whereas they are usually lower for smaller systems. Energy costs are greater with the membrane process due to the high pumping volume required to achieve turbulent-flow velocities across the membrane surface to maximize flux rate and minimize fouling
Table 2 summarizes some of the main differences between the two processes. For total treatment systems with water sources requiring pretreatment to control slightly soluble salts (including Ca, Mg, Si and heavy metals), this membrane technology offers significant advantages.
Total treatment systems
In many applications, the pretreated water needs “polishing” to remove salts or low-molecular-weight organic contaminants that cannot be removed with MF/UF technologies.
The most common polishing technology is RO, and as previously mentioned, this process most frequently utilizes spiral-wound membrane elements (Figure 1). This construction is the least expensive in terms of membrane area. However, it cannot be backwashed to remove foulants from the membrane surface. The tubular MF/UF membranes discussed previously can be readily backwashed. This characteristic alone makes tubular MF/UF membranes invaluable for many applications, such as wastewater recovery and reuse. In many of these applications, the wastewater contains high concentrations of suspended solids and dissolved organics that are capable of irreversibly fouling RO membranes.
As stated previously, the utilization of membrane technology for the treatment (and concentration) of pH-adjusted industrial wastewater streams is virtually the same as for groundwater sources (raise the pH, treat with tubular membranes and dewater the concentrate stream). Therefore, this treatment approach is effective over a wide range of applications.
A practical example
The authors both have many years of experience in the design, construction and operation of wastewater recovery and reuse systems utilizing membrane technologies. For example, they recently consulted in the design and construction of a total treatment system originally designed to treat well water for a large recreational facility utilizing an inefficient treatment system. The new system treated the wastewater discharge from the original system by recovering the precipitated hardness salts with MF technology and a filter press, polishing the MF permeate with RO and employing crystallization and evaporation technologies to produce dry solids for landfilling — the result was a zero liquid discharge (ZLD) system. ■
Edited by Mary Page Bailey
Authors
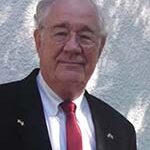
Peter S. Cartwright
Peter S. Cartwright (Website: www.cartwright-consulting.com; Email: [email protected]) has been in the water and wastewater treatment industry since 1974 and has had his consulting engineering business since 1980. He has written many articles and book chapters and has presented over 300 lectures throughout the world. Cartwright is active in a number of technical societies and is a licensed Professional Engineer in Pennsylvania.
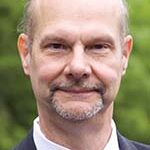
Doug Frick
Doug Frick (Email: [email protected]) is a 25-year veteran of the water and wastewater business, and has worked mainly with industrial wastewater processes and membrane filtration. He is holds a degree in geological engineering from the University of Minnesota and has traveled worldwide for customers, providing training and reviewing hundreds of facilities and sites. He is a licensed Professional Geologist.